Lab 10: Cardiovascular System I – Blood and the Heart
Blood tissue and the Heart
Learning Objectives
When you are prepared for the Test on Week 10 Learning Objectives in Week 11, you will be able to:
- Differentiate between red blood cells, white blood cells, and platelets in appearance and general functions.
- Differentiate between white blood cell types in terms of appearance, proportion in a healthy individual, and how they are used to diagnose various health conditions.
- Identify structures of the heart and their functions.
- Identify major blood vessels going in and out of the heart and coronary blood vessels.
- Describe the pathway of blood through the heart and associated blood vessels, and state whether the blood is oxygenated or deoxygenated at any point in that pathway.
Blood
Blood is a connective tissue made up of cellular elements and an extracellular matrix. The cellular elements are referred to as the formed elements and include red blood cells (RBCs), white blood cells (WBCs), and platelets. The extracellular matrix, called plasma, makes blood unique among connective tissues because it is fluid. This fluid, which is mostly water, perpetually suspends the formed elements and enables them to circulate throughout the body within the cardiovascular system.In the laboratory, blood samples are often centrifuged in order to separate the components of blood from one another (Figure 10.1). Erythrocytes are the heaviest elements in blood and settle at the very bottom of the tube. Above the erythrocyte layer we see the buffy coat, a pale, thin layer of leukocytes and platelets, which together make up less than 1% of the sample of whole blood. Above the buffy coat is the blood plasma, normally a pale, straw-colored fluid, which constitutes the remainder of the sample.
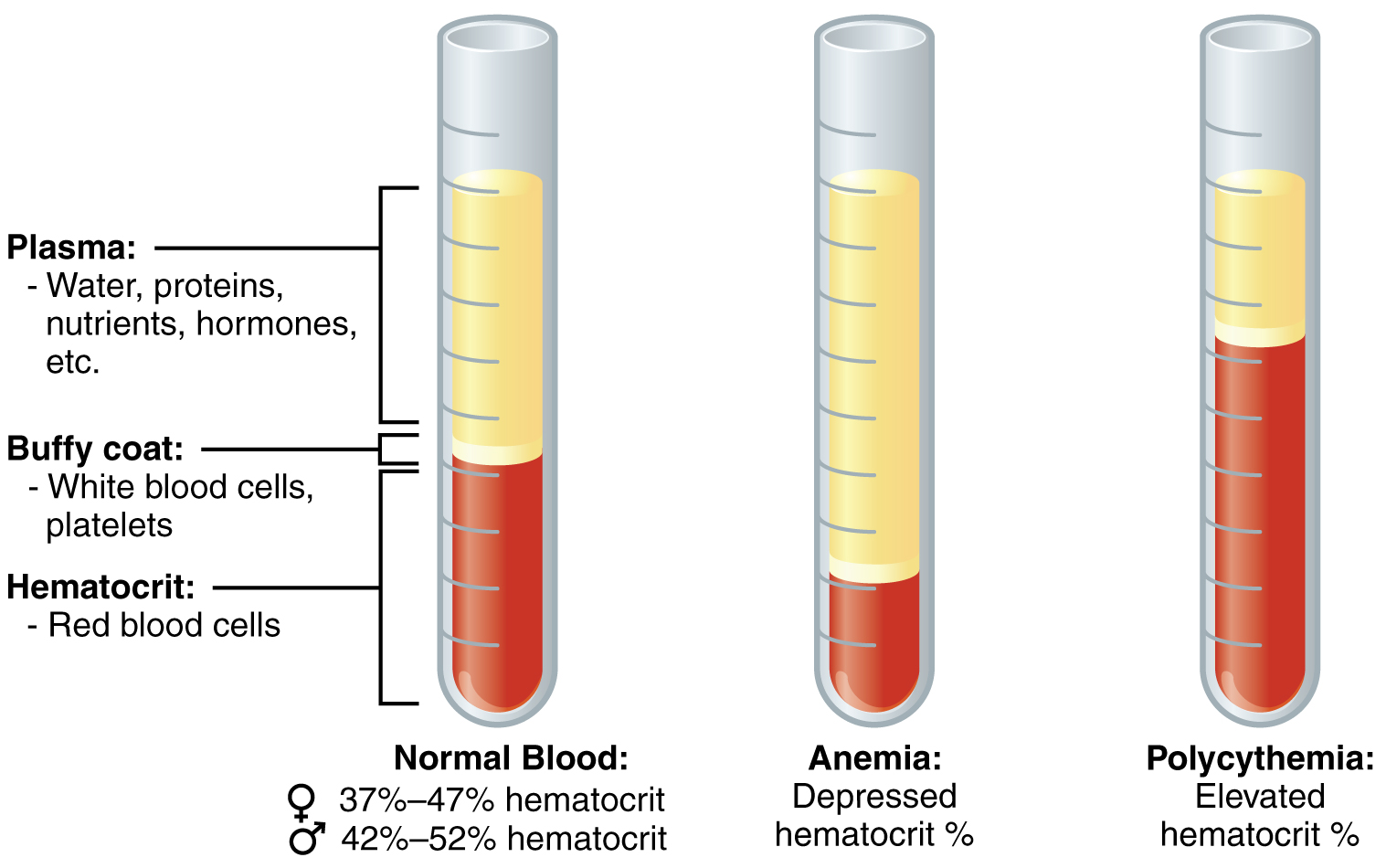
In normal blood, about 45 percent of a sample is erythrocytes, which is referred to as the hematocrit. The hematocrit of any one sample can vary significantly, however, about 36–50 percent, according to gender and other factors. Not counting the buffy coat, which makes up less than 1% of the blood, we can estimate the mean plasma percentage to be the percent of blood that is not erythrocytes: approximately 55% (Table 10.1).
COMPONENT AND % OF BLOOD | SUBCOMPONENT AND % OF COMPONENT | TYPE AND % (WHERE APPROPRIATE) | SITE OF PRODUCTION | MAJOR FUNCTION(S) |
Plasma
46 – 63% of blood |
Water; 92% of plasma | Fluid | Absorbed by intestinal tract or produced by metabolism | Transport medium |
Plasma proteins; 7% of plasma | Albumin; 54 – 60% of plasma proteins | Liver | Maintain osmotic concentration, transport lipid molecules | |
Globulins; 35 – 38% of plasma proteins | Alpha globulins – liver | Transport, maintain osmotic concentration | ||
Beta globulins – liver | Transport, maintain osmotic concentration | |||
Gamma globulins (immunoglobulins) – plasma cells | Immune responses | |||
Fibrinogen 4 – 7 percent | Liver | Blood clotting in hemostasis | ||
Regulatory proteins; <1% of plasma | Hormones and enzymes | Various sources | Regulate various body functions | |
Other solutes; 1% of plasma | Nutrients, gases, and wastes | Absorbed by intestinal tract, exchanged in respiratory system, or produced by cells | Numerous and varied | |
Formed elements
37 – 54% of blood |
Erythrocytes; 99% of formed elements | Erythrocytes | Red bone marrow | Transport gases, primarily oxygen and some carbon dioxide |
Leukocytes; <1% of formed elements | Granular Leukocytes: neutrophils eosinophils basophils |
Red bone marrow | Nonspecific immunity | |
Agranular leukocytes: lymphocytes monocytes |
Lymphocytes: bone marrow and lymphatic tissue | Lymphocytes: specific immunity |
||
Monocytes: red bone marrow | Monocytes: nonspecific immunity |
|||
Platelets; <1% of formed elements | n/a | Megakaryocytes: Red Bone Marrow | Hemostasis |
Functions of Blood
Although carrying oxygen and nutrients to cells and removing wastes from cells is the main function of blood, it is important to realize that blood also serves in defense, distribution of heat, and maintenance of homeostasis.
Transportation
- Nutrients from the foods you eat are absorbed in the digestive tract. Most of these travel in the bloodstream directly to the liver, where they are processed and released back into the bloodstream for delivery to body cells.
- Oxygen from the air you breathe diffuses into the blood, which moves from the lungs to the heart, which then pumps it out to the rest of the body.
- Endocrine glands scattered throughout the body release their products, called hormones, into the bloodstream, which carries them to distant target cells.
- Blood also picks up cellular wastes and byproducts, and transports them to various organs for removal. For instance, blood moves carbon dioxide to the lungs for exhalation from the body, and various waste products are transported to the kidneys and liver for excretion from the body in the form of urine or bile.
Defense
- Leukocytes protect the organism from disease-causing bacteria, cells with mutated DNA that could multiply to become cancerous, or body cells infected with viruses.
- When damage to the vessels results in bleeding, blood platelets and certain proteins dissolved in the plasma, interact to block the ruptured areas of the blood vessels involved. This protects the body from further blood loss.
Homeostasis
- If you were exercising on a warm day, your rising core body temperature would trigger several homeostatic mechanisms, including increased transport of blood from your core to your body periphery, which is typically cooler. As blood passes through the vessels of the skin, heat would be dissipated to the environment, and the blood returning to your body core would be cooler. In contrast, on a cold day, blood is diverted away from the skin to maintain a warmer body core. In extreme cases, this may result in frostbite.
- Blood helps to regulate the water content of body cells.
- Blood also helps to maintain the chemical balance of the body. Proteins and other compounds in blood act as buffers, which thereby help to regulate the pH of body tissues. The pH of blood ranges from 7.35 to 7.45.
Components of Blood
Blood Plasma
Like other fluids in the body, plasma is composed primarily of water. In fact, it is about 92% water. Dissolved or suspended within this water is a mixture of substances, most of which are proteins. The major components of plasma and their functions are summarized in the table above.
Formed Elements
The formed elements of blood include red blood cells (RBCs), white blood cells (WBCs), and platelets. Table 10.2 summarizes the main facts about the formed elements in blood.
FORMED ELEMENT | NUMBER PRESENT PER MICROLITER (µL) AND MEAN (RANGE) | APPEARANCE IN A STANDARD BLOOD SMEAR | SUMMARY OF FUNCTIONS | COMMENTS |
Erythrocytes (red blood cells)
![]() |
5.2 million ( 4.4-5.0 million) | Flattened biconcave disk; no nucleus; pale red colour | Transport oxygen and some carbon dioxide between tissues and lungs | Lifespan of approximately 120 days |
Leukocytes (white blood cells)
|
7000 (5000 – 10,000) | Obvious dark-staining nucleus | All function in body defenses | Exit capillaries and move into tissues; lifespan of usually a few hours or days |
Platelets
![]() |
350,000 (150,000 – 500,000) | Cellular fragments surrounded by a plasma membrane and containing granules; purple stain | Hemostasis plus release growth factors for repair and healing of tissue | Formed from megakaryocytes that remain in the red bone marrow and shed platelets into circulation |
Erythrocytes
The most abundant formed elements in blood, erythrocytes, are basically sacs packed with an oxygen-carrying compound called hemoglobin. They are flattened, biconcave discs and relatively small, which allows them to travel through small-diameter capillaries. The primary functions of erythrocytes are to pick up inhaled oxygen from the lungs and transport it to the body’s tissues. At maturity, erythrocytes lose their nucleus and most of their organelles, which means there is more interior space for the presence of the hemoglobin molecules that transport gases. The biconcave shape also provides a greater surface area across which gas exchange can occur, relative to its volume; a sphere of a similar diameter would have a lower surface area-to-volume ratio (Figure 10.2).
Production of erythrocytes in the red bone marrow occurs at the staggering rate of more than 2 million cells per second. For this production to occur, raw materials including iron, copper, zinc, B-vitamins, glucose, lipids, and amino acids must be present in adequate amounts. Erythrocytes live only 120 days on average, and thus must be continually replaced. Worn-out erythrocytes are phagocytized by macrophages and their hemoglobin is broken down. The breakdown products are recycled or removed as wastes.
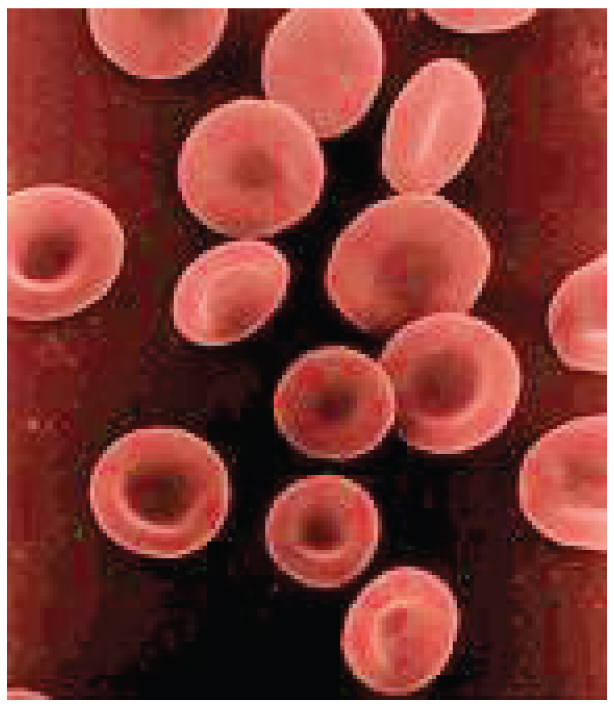
Changes in the levels of RBCs can have significant effects on the body’s ability to effectively deliver oxygen to the tissues. The size, shape, and number of erythrocytes, and the number of hemoglobin molecules can have a major impact on a person’s health. When the number of RBCs or hemoglobin is deficient, the general condition is called anemia. There are more than 400 types of anemia.
Anemia can be broken down into three major groups: those caused by blood loss, those caused by excessive destruction of RBCs, and those caused by faulty or decreased RBC production. Of those types of anemia caused by faulty or decreased RBC production, the most common type is iron deficiency anemia, which results when the amount of available iron is insufficient to allow production of sufficient hemoglobin. Another dietary deficiency that can lead to various types of anemia is insufficient vitamin B12 and/or folate. Faulty RBC production can be caused by sickle cell anemia, a genetic disorder involving the production of an abnormal type of hemoglobin which delivers less oxygen to tissues and causes erythrocytes to assume a sickle (or crescent) shape (Figure 10.3).
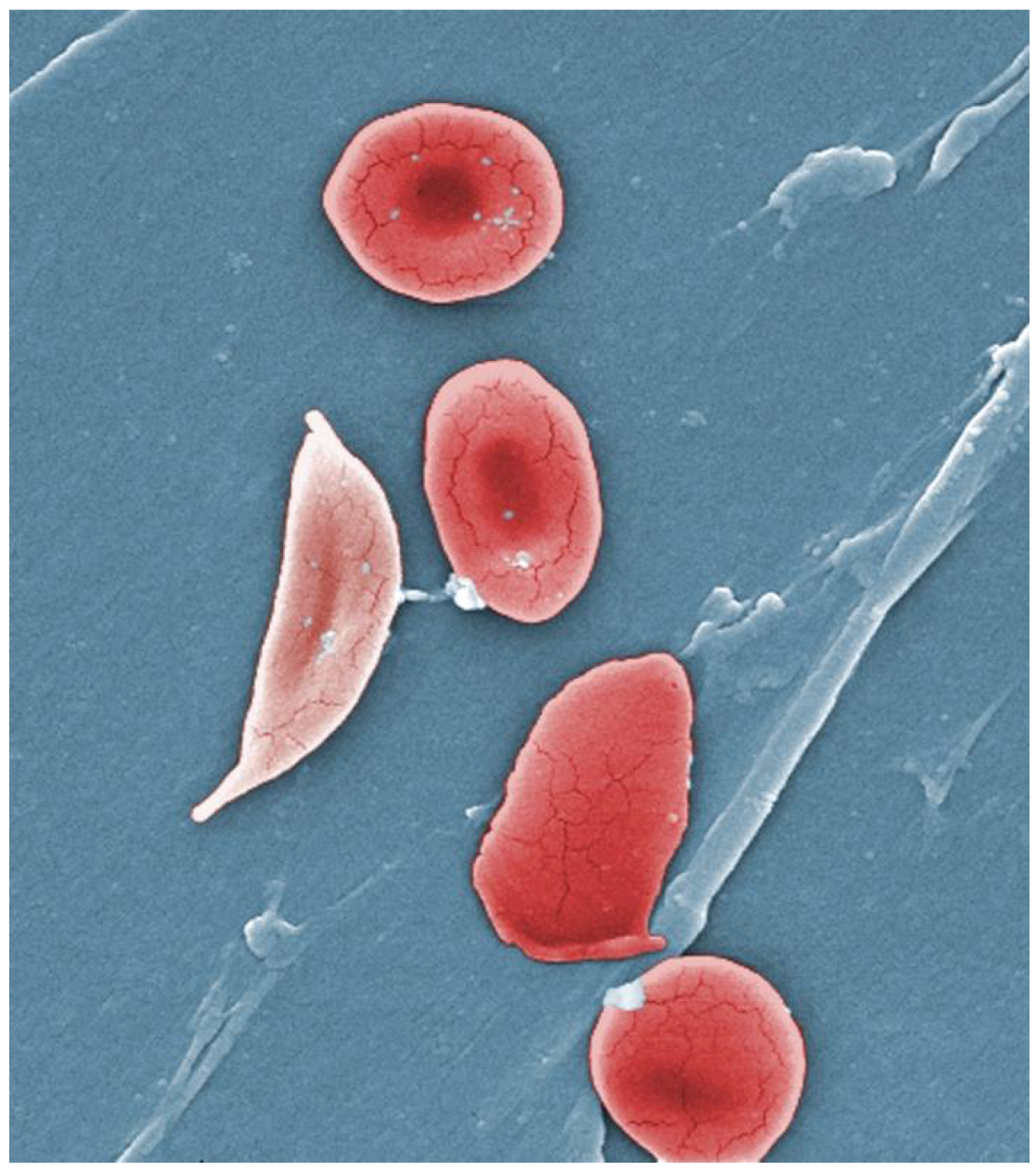
In addition to these causes, various disease processes also can lead to anemias. These include chronic kidney diseases often associated with a decreased production of EPO (a hormone that signals RBC production), hypothyroidism, some forms of cancer, lupus, and rheumatoid arthritis.
Polycythemia is an elevated RBC count. It can occur transiently in a person who is dehydrated; when water intake is inadequate or water losses are excessive, the plasma volume falls. As a result, the hematocrit rises. A mild form of polycythemia is chronic but normal in people living at high altitudes. Some elite athletes train at high elevations specifically to induce this phenomenon. Finally, a type of bone marrow disease called polycythemia vera causes an excessive production of immature erythrocytes. Polycythemia vera can dangerously elevate the viscosity of blood, raising blood pressure and making it more difficult for the heart to pump blood throughout the body. It is a relatively rare disease that occurs more often in men than women, and is more likely to be present in elderly patients those over 60 years of age.
Leukocytes
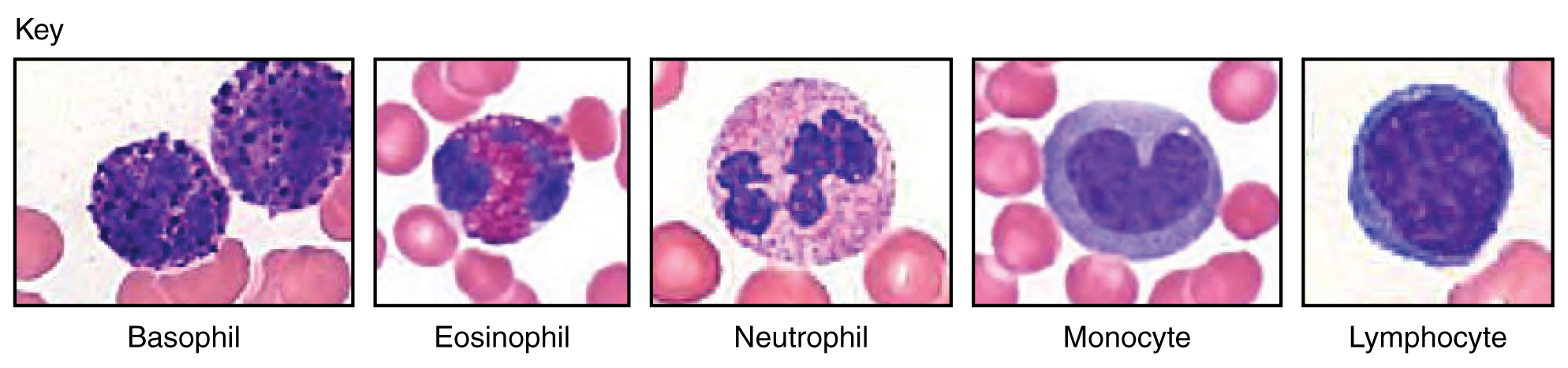
When scientists first began to observe stained blood slides, it quickly became evident that leukocytes could be divided into two groups, according to whether their cytoplasm contained highly visible granules:
- Granular leukocytes contain abundant granules within the cytoplasm. They typically have a lobed nucleus and classified by which type of stain best highlights their granules. They include neutrophils, eosinophils, and basophils.
- While granules are not totally lacking in agranular leukocytes, they are far fewer and less obvious. Agranular leukocytes include monocytes, which mature into macrophages that are phagocytic, and lymphocytes, which arise from the lymphoid stem cell line.
Each white blood cell type has a distinctive appearance and fulfills a different role in the immune system response (Table 10.3). If a pathogen is detected, the body increases production of the WBC type(s) that are specialized for that particular pathogen. Because of this, counting relative abundances of WBCs in a patient and comparing them to the typical abundance you would expect in a healthy individual can be useful during diagnosis.
Name | Type |
Typical Abundance in a WBC count | Appearance | Functions | Conditions related to high counts | Notes |
Neutrophils
|
Granulocytes | 50-70% | – “Neutral” (pale lilac) colored granules
– Light purple cytoplasm – Most segmented nucleus (2-5 lobes) |
Phagocytic; particularly effective against bacteria. Release cytotoxic chemicals from granules | Infection (particularly bacterial), inflammation, burns, unusual stress | Most common leukocyte; lifespan of minutes to days |
Eosinophils
|
1-4% | – Dark pink/orange/red granules
– Bi-lobed nucleus |
Phagocytic cells; particularly effective with antigen-antibody complexes. Release antihistamines. Increase in allergies and parasitic infections | Allergies, parasitic worm infestations, some autoimmune diseases | Lifespan of minutes to days | |
Basophils
|
0.5-1% | – Dark blue/purple granules that obscure nucleus and cytoplasm
– Bi-lobed nucleus |
Promotes inflammation; Release histamines and heparin during allergic reactions | Allergies, hypothyroidism | Least common leukocyte; lifespan unknown | |
Lymphocytes
|
Agranulocytes | 20-40% | -Spherical cells with a single, often large nucleus occupying much of the cell’s volume
-stains purple -see in large (natural killer cells) and small (B and T cells) variants |
Attack pathogens and virus-infected cells; coordinate body’s immune response. T cells directly attack other cells (cellular immunity). B cells release antibodies (humoral immunity); natural killer cells are similar to T cells but nonspecific | Viral infections, some cancers | Initial cells originate in bone marrow, but secondary production occurs in lymphatic tissue; several distinct subtypes; memory cells form after exposure to a pathogen and rapidly increase responses to subsequent exposure; lifespan of many years |
Monocytes
|
2-8% | -Largest leukocyte (2-3x size of RBC)
-indented or horseshoe-shaped nucleus |
Very effective phagocytic cells engulfing pathogens or worn out cells; also serve as antigen-presenting cells (APCs) for other components of the immune system | Viral or fungal infections, tuberculosis, some forms of leukemia, other chronic diseases | Produced in red bone marrow; referred to as macrophages after leaving circulation |
Leukocytes routinely leave the bloodstream to perform their defensive functions in the body’s tissues, where they are often given distinct names, such as macrophage or microglia, depending on their function. They leave the capillaries—the smallest blood vessels—or other small vessels through a process known as emigration or diapedesis in which they squeeze through adjacent cells in a blood vessel wall (Figure 10.5).
Once they have exited the capillaries, some leukocytes will take up fixed positions in lymphatic tissue, bone marrow, the spleen, the thymus, or other organs. Others will move about through the tissue spaces, sometimes wandering freely, and sometimes moving toward the direction in which they are drawn by chemical signals.
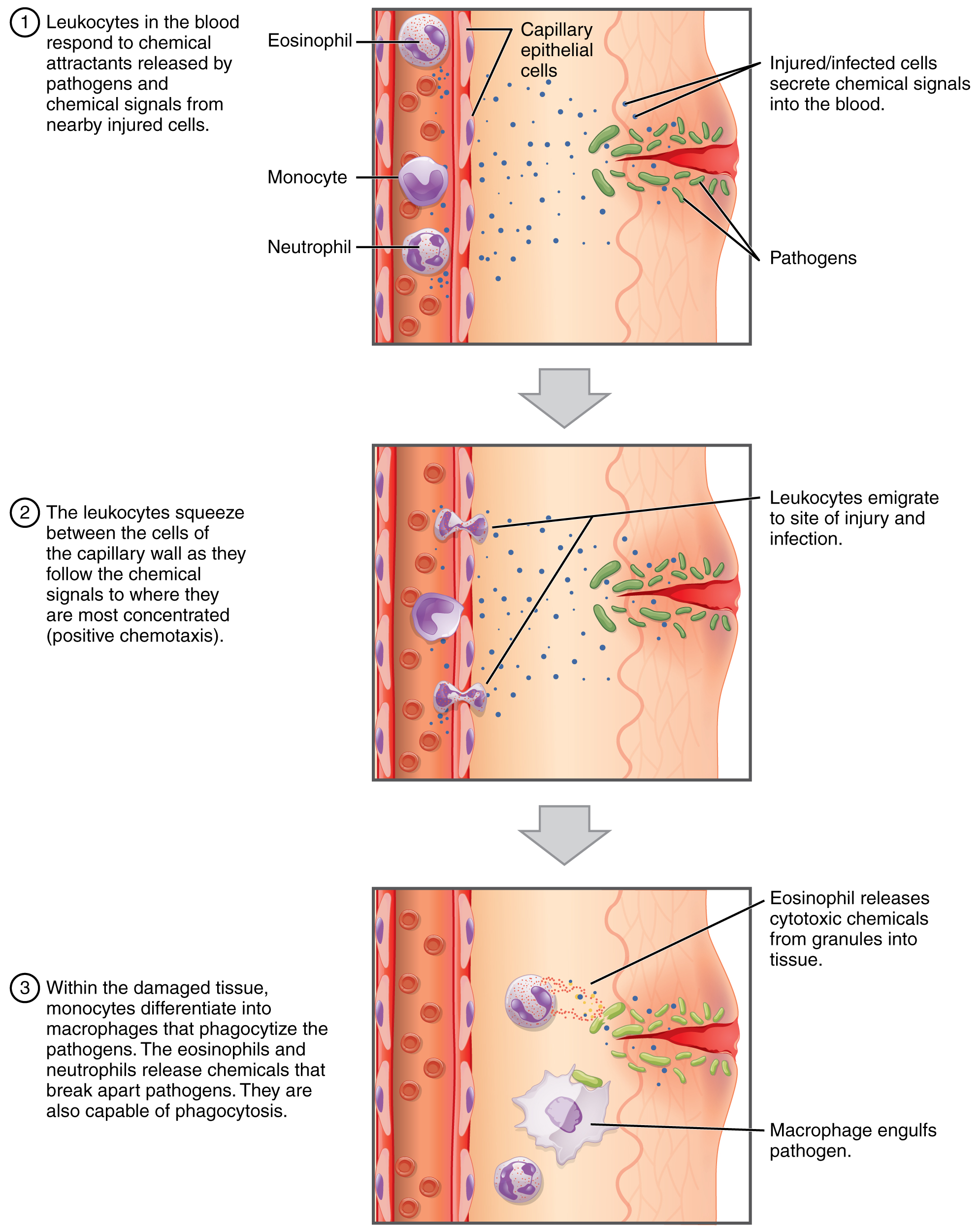
Platelets
A platelet is not a cell but rather a fragment of the cytoplasm of a cell called a megakaryocyte that is surrounded by a plasma membrane. Platelets are much smaller than RBCs, but numerous. After entering the circulation, approximately one-third of the newly-formed platelets migrate to the spleen for storage for later release in response to any rupture in a blood vessel. They then become activated to perform their primary function, which is to limit blood loss. Platelets remain only about 10 days, then are phagocytized by macrophages.
Platelets are key players in hemostasis, the process by which the body seals a ruptured blood vessel and prevents further loss of blood. Although rupture of larger vessels usually requires medical intervention, hemostasis is quite effective in dealing with small, simple wounds. There are three steps to the process: vascular spasm, the formation of a platelet plug, and coagulation (blood clotting). Failure of any of these steps will result in hemorrhage.
Hemopoiesis/Hematopoiesis
The lifespan of the formed elements is very brief. Although one type of leukocyte (memory cells) can survive for years, most erythrocytes, leukocytes, and platelets normally live only a few hours to a few weeks. Thus, the body must form new blood cells and platelets quickly and continuously, a process known as hemopoiesis, or hematopoiesis. In children, hemopoiesis can occur in the medullary cavity of long bones; in adults, the process is largely restricted to the red bone marrow in the cranial and pelvic bones, the vertebrae, the sternum, and the proximal epiphyses of the femur and humerus.
All formed elements arise from stem cells of the red bone marrow, called a hemopoietic stem cell, or hemocytoblast (Figure 10.6).
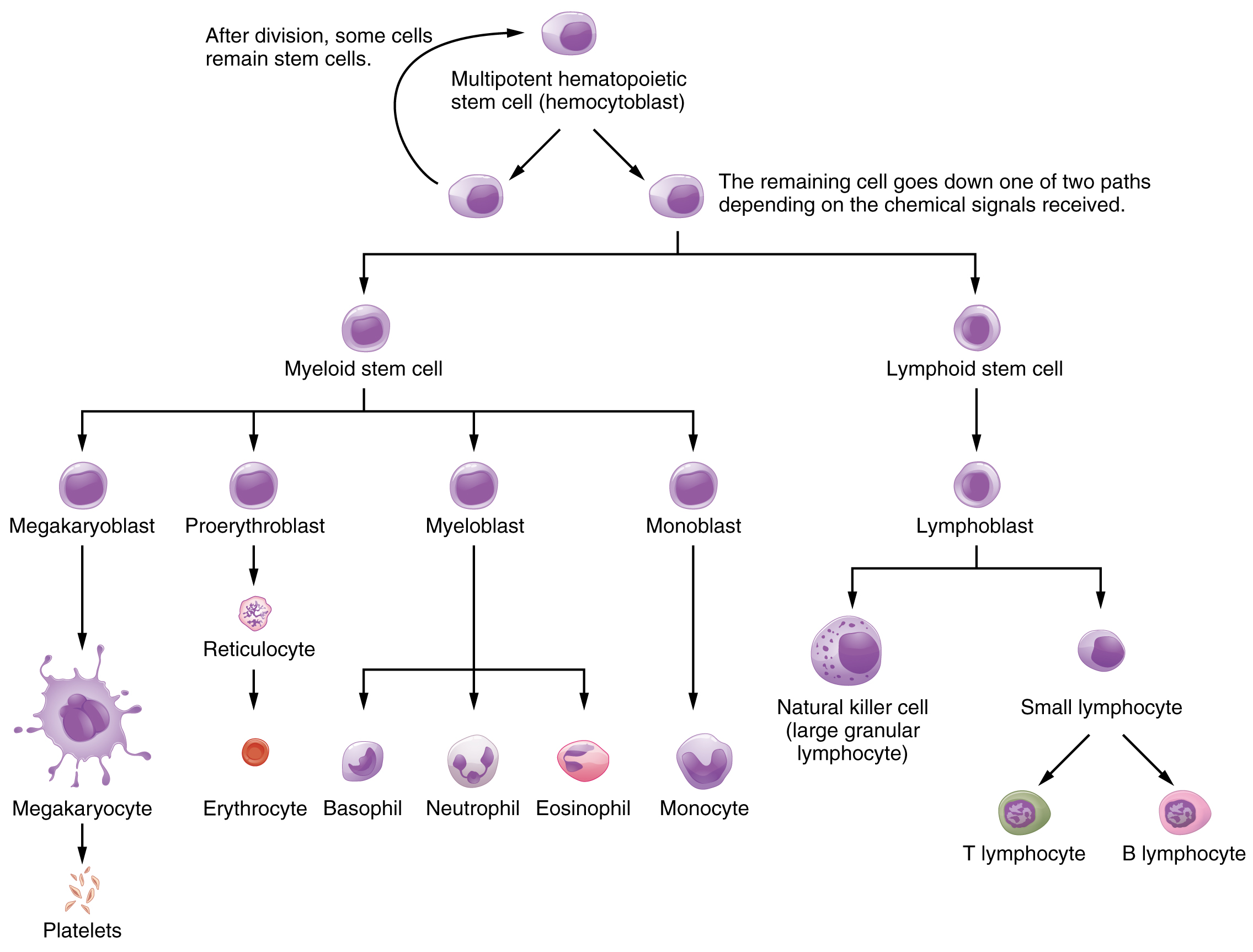
The Heart
The heart is a fist-sized vital organ that has one job: to pump blood. If one assumes an average heart rate of 75 beats per minute, a human heart would beat approximately 108,000 times in one day, more than 39 million times in one year, and nearly 3 billion times during a 75-year lifespan. At rest, each of the major pumping chambers of the heart ejects approximately 70 mL blood per contraction in an adult. This would be equal to 5.25 liters of blood per minute and approximately 14,000 liters per day. Over one year, that would equal 10,000,000 liters of blood sent through roughly 100,000 km of blood vessels. In order to understand how that happens, it is necessary to understand the anatomy and physiology of the heart.
Anatomy of the Heart
Location
The human heart is located within the thoracic cavity, between the lungs in the space known as the mediastinum (Figure 10.7). Within the mediastinum, the heart is separated from the other mediastinal structures by a tough membrane known as the pericardium, or pericardial sac, and sits in its own space called the pericardial cavity. The great vessels, which carry blood to and from the heart, are attached to the superior surface of the heart, which is called the base. The base of the heart is located at the level of the third costal cartilage. The inferior tip of the heart, the apex, lies just to the left of the sternum between the junction of the fourth and fifth ribs.
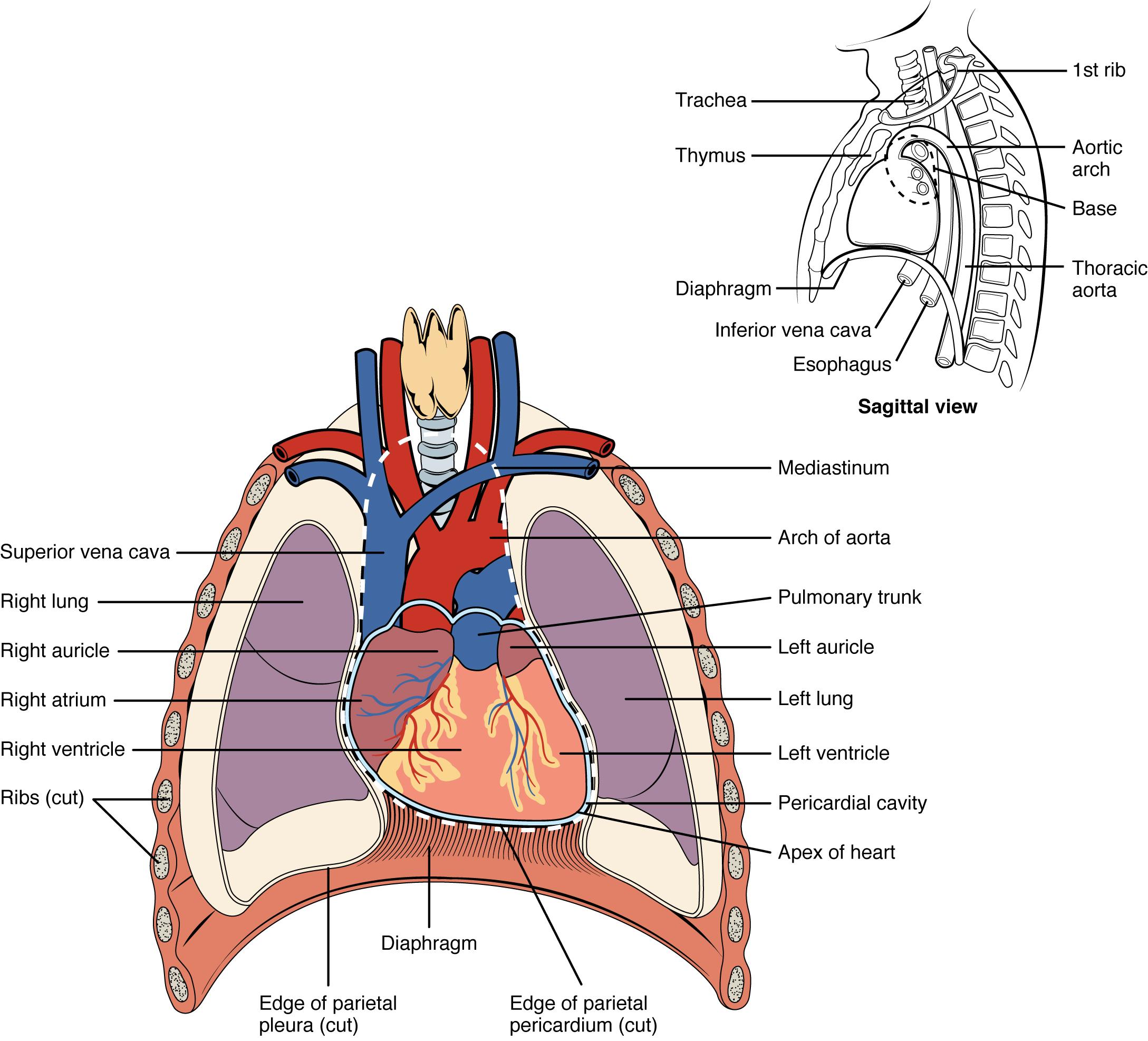
Membranes and Layers of the Heart Walls
The heart and the roots of the great vessels are surrounded by a membrane known as the pericardium or pericardial sac. The pericardium consists of two distinct sub layers (Figure 10.9):
- The sturdy outer fibrous pericardium is made of tough, dense connective tissue that protects the heart and holds it in position.
- Separated by the pericardial cavity and containing pericardial fluid the inner serous pericardium consists of two layers:
- the outer parietal pericardium, which is fused to the fibrous pericardium.
- the inner visceral pericardium, or epicardium, which is fused to the heart and forms the outer layer of the heart wall.
The walls of the heart consist of three layers (Figure 10.8):
- The outer epicardium, which is another name for the visceral pericardium mentioned above.
- The thick, middle myocardium, which is made of muscle tissue and gives the heart its ability to contract.
- The inner endocardium, which lines the heart chambers and is the main component of the heart valves.
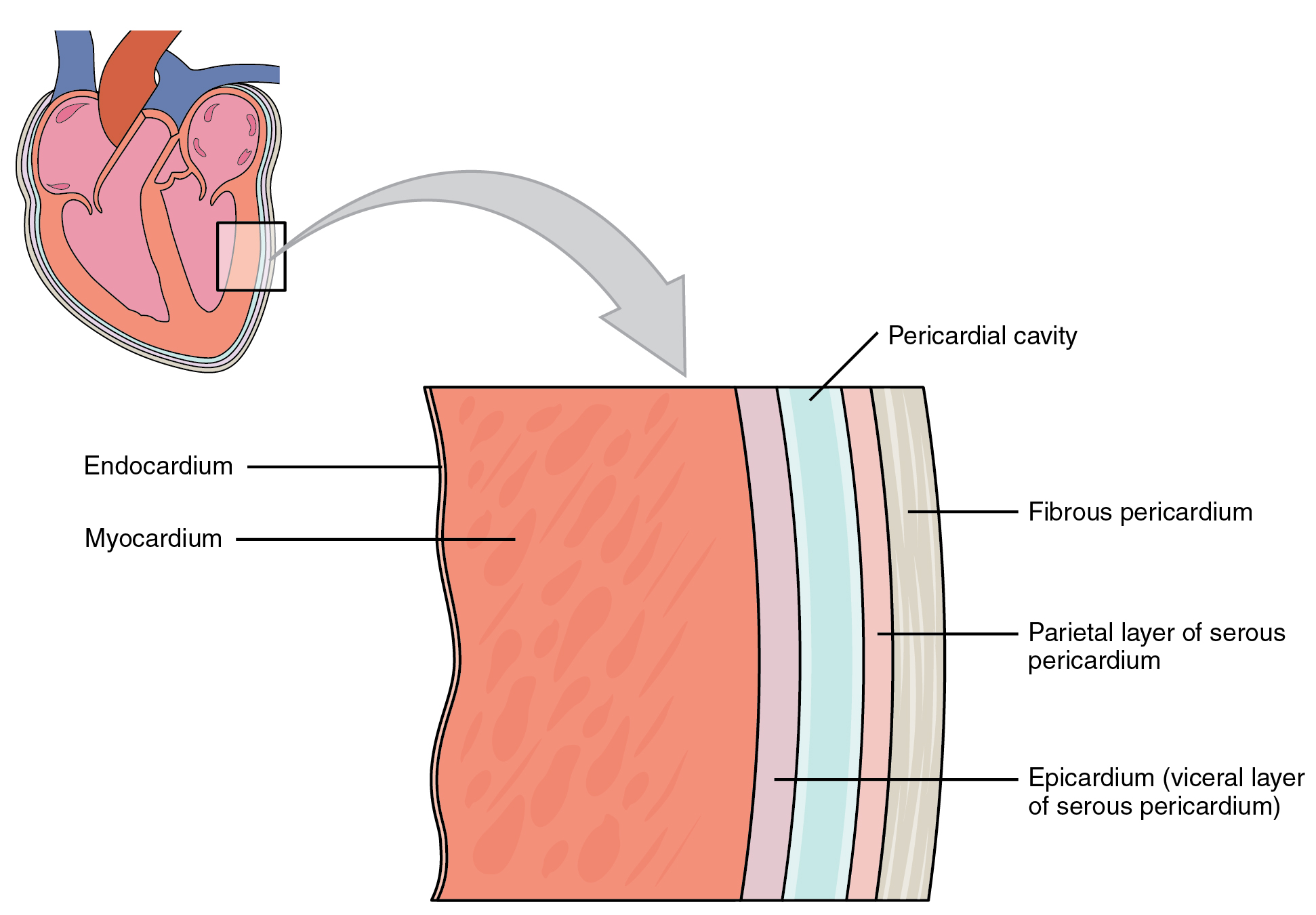
Surface Features of the Heart
Inside the pericardium, the surface features of the heart are visible, including the four chambers. There is a superficial leaf-like extension of the atria near the superior surface of the heart, one on each side, called an auricle—a name that means “ear like”—because its shape resembles the external ear of a human (Figure 10.9). Auricles are relatively thin-walled structures that can fill with blood and empty into the atria or upper chambers of the heart. You may also hear them referred to as atrial appendages. Also prominent is a series of fat-filled grooves, each of which is known as a sulcus (plural = sulci), along the superior surfaces of the heart. Major coronary blood vessels are located in these sulci. The deep coronary sulcus is located between the atria and ventricles. Located between the left and right ventricles are two additional sulci that are not as deep as the coronary sulcus. The anterior interventricular sulcus is visible on the anterior surface of the heart, whereas the posterior interventricular sulcus is visible on the posterior surface of the heart. Figure 10.9 illustrates anterior and posterior views of the surface of the heart.
Internal Structures of the Heart
The human heart consists of four chambers: The left side and the right side each have one atrium and one ventricle. Each of the upper chambers, the right atrium (plural = atria) and the left atrium, acts as a receiving chamber and contracts to push blood into the lower chambers, the right ventricle and the left ventricle. The ventricles serve as the primary pumping chambers of the heart, propelling blood to the lungs or to the rest of the body. The majority of the internal heart structures discussed in this section are illustrated in Figure 10.10.
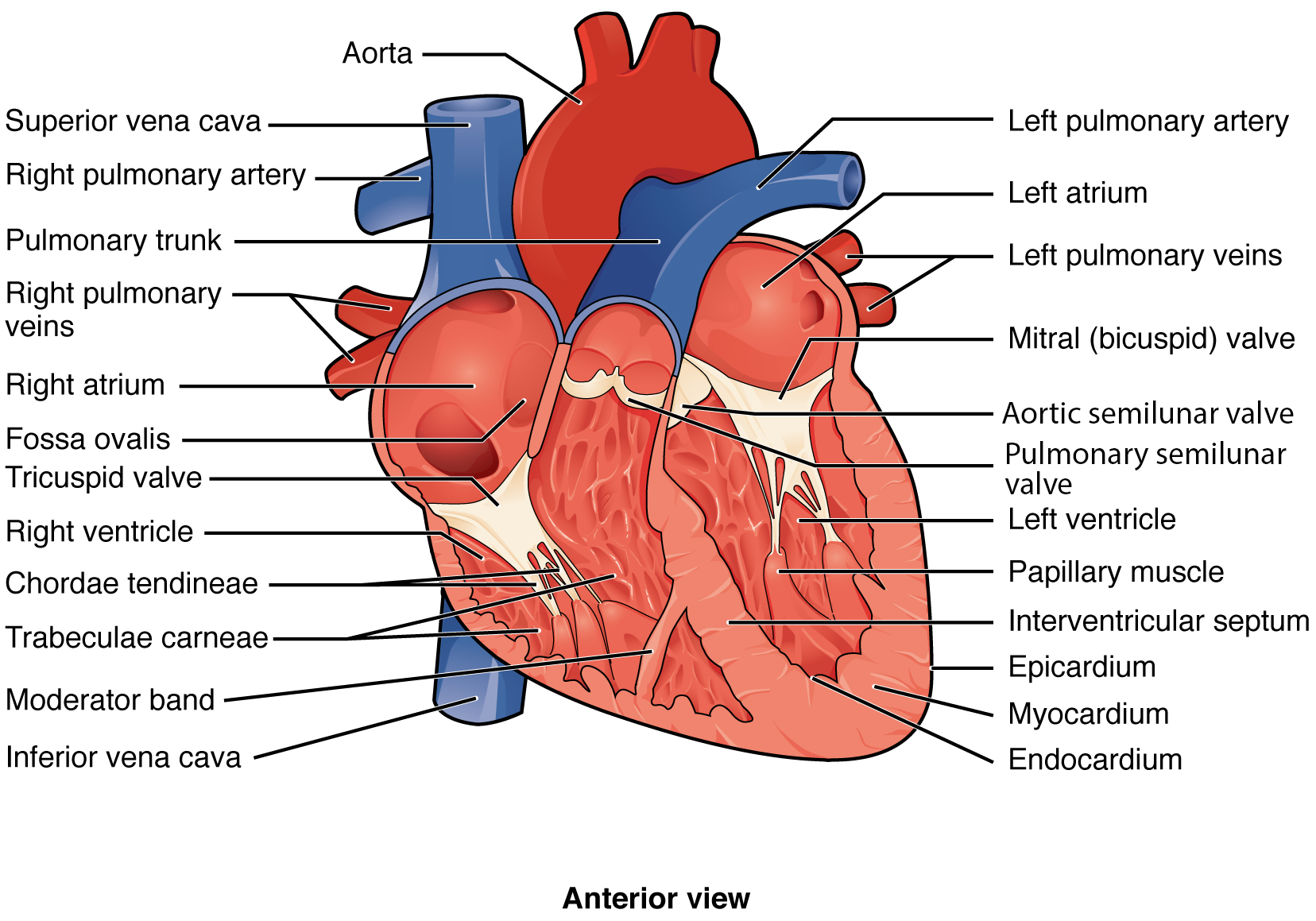
The right atrium is separated from the left atrium by a wall called the interatrial septum. Normally in an adult heart, the interatrial septum bears an oval-shaped depression known as the fossa ovalis, a remnant of an opening in the fetal heart known as the foramen ovale. The foramen ovale allowed blood in the fetal heart to pass directly from the right atrium to the left atrium, allowing some blood to bypass the pulmonary circuit. Between the two ventricles is a second septum known as the interventricular septum. It is substantially thicker than the interatrial septum, since the ventricles generate far greater pressure when they contract.
The septum between the atria and ventricles is known as the atrioventricular septum. It is marked by the presence of four openings that allow blood to move from the atria into the ventricles and from the ventricles into the pulmonary trunk and aorta. Located in each of these openings between the atria and ventricles is a valve, a specialized structure that ensures one-way flow of blood. The valves between the atria and ventricles are known generically as atrioventricular valves. The valves at the openings that lead to the pulmonary trunk and aorta are known generically as semilunar valves. A transverse section through the heart slightly above the level of the atrioventricular septum reveals all four heart valves along the same plane (Figure 10.11).
Right atrium
The right atrium serves as the receiving chamber for blood returning to the heart from the systemic circulation. The two major systemic veins, the superior and inferior venae cavae, and the large coronary vein called the coronary sinus that drains the heart myocardium empty into the right atrium.
While the bulk of the internal surface of the right atrium is smooth, the depression of the fossa ovalis is medial, and the anterior surface demonstrates prominent ridges of muscle called the pectinate muscles. The right auricle also has pectinate muscles. The left atrium does not have pectinate muscles except in the auricle. The right atrioventricular valve, or tricuspid valve, separates the right atrium and right ventricle.
Right ventricle
The right ventricle receives blood from the right atrium through the tricuspid valve. Each flap of the valve is attached to strong strands of connective tissue, the chordae tendineae, literally “tendinous cords,” or sometimes more poetically referred to as “heart strings.” They connect each of the flaps to a papillary muscle that extends from the inferior ventricular surface. There are three papillary muscles in the right ventricle, called the anterior, posterior, and septal muscles, which correspond to the three sections of the valves.
When the myocardium of the ventricle contracts, pressure within the ventricular chamber rises. Blood, like any fluid, flows from higher pressure to lower pressure areas, in this case, toward the pulmonary trunk and the atrium. To prevent any potential backflow, the papillary muscles also contract, generating tension on the chordae tendineae. This prevents the flaps of the valves from being forced into the atria and regurgitation of the blood back into the atria during ventricular contraction. Figure 10.12 shows papillary muscles and chordae tendineae attached to the tricuspid valve.
The walls of the ventricle are lined with trabeculae carneae, ridges of cardiac muscle covered by endocardium. When the right ventricle contracts, it ejects blood into the pulmonary trunk, which branches into the left and right pulmonary arteries that carry it to each lung. At the base of the pulmonary trunk is the pulmonary semilunar valve that prevents backflow from the pulmonary trunk.
Left Atrium
After exchange of gases in the pulmonary capillaries, blood returns to the left atrium high in oxygen via one of the four pulmonary veins. Blood flows nearly continuously from the pulmonary veins back into the atrium, which acts as the receiving chamber, and from here through an opening into the left ventricle.
The left atrioventricular valve, or bicuspid valve, separates the left atrium and left ventricle. This valve is also called the mitral valve. In Figure 10.13a, the two atrioventricular valves are open and the two semilunar valves are closed. This occurs when both atria and ventricles are relaxed and when the atria contract to pump blood into the ventricles. Figure 10.13b shows a frontal view. Although only the left side of the heart is illustrated, the process is virtually identical on the right.
Left ventricle
The left ventricle receives blood from the left atrium through the bicuspid valve. Like in the right ventricle, the bicuspid valve is connected to papillary muscles via chordae tendineae to prevent prolapse. There are two papillary muscles on the left—the anterior and posterior—as opposed to three on the right.
The left ventricle is the major pumping chamber for the systemic circuit; it ejects blood into the aorta through the aortic semilunar valve.
Although the ventricles on the right and left sides pump the same amount of blood per contraction, the muscle of the left ventricle is much thicker and better developed than that of the right ventricle. In order to overcome the high resistance required to pump blood into the long systemic circuit, the left ventricle must generate a great amount of pressure. The right ventricle does not need to generate as much pressure, since the pulmonary circuit is shorter and provides less resistance. Figure 10.14 illustrates the differences in muscular thickness needed for each of the ventricles.
The Coronary Blood Supply
Myocardial cells require their own blood supply to carry out their function of contracting and relaxing the heart in order to pump blood. Their own blood supply provides nutrients and oxygen and carry away carbon dioxide and waste. These functions are provided by the coronary arteries and coronary veins.
Coronary arteries supply blood to the myocardium and other components of the heart (Figure 10.15). The first portion of the aorta after it arises from the left ventricle gives rise to the left and right coronary arteries. The left coronary artery distributes blood to the left side of the heart, the left atrium and ventricle, and the interventricular septum. The circumflex artery arises from the left coronary artery and follows the coronary sulcus to the left. The larger anterior interventricular artery, also known as the left anterior descending artery (LAD), is the second major branch arising from the left coronary artery. It follows the anterior interventricular sulcus. The right coronary artery proceeds along the coronary sulcus and distributes blood to the right atrium, portions of both ventricles, and the heart conduction system. Smaller arteries branch off of these main ones so that all of the heart wall is supplied.
Coronary veins drain the heart and generally parallel the large surface arteries. These veins drain the areas of the heart supplied by the coronary arteries and bring the deoxygenated blood to the coronary sinus on the posterior surface of the heart. The coronary sinus is a large, thin-walled vein on the posterior surface of the heart lying within the atrioventricular sulcus and emptying directly into the right atrium.
Pathway of Blood Through the Heart
The process of pumping and circulating blood is active, coordinated and rhythmic. Each heartbeat represents one cycle of the heart receiving blood and ejecting blood. The heart pumps blood to two distinct but linked circulatory systems called the pulmonary and systemic circuits. The pulmonary circuit transports blood to and from the lungs, where it picks up oxygen and drops off carbon dioxide. The systemic circuit transports freshly oxygenated blood to virtually all of the tissues of the body and returns relatively deoxygenated blood and carbon dioxide to the heart to be sent back to the pulmonary circulation (Figure 10.16).
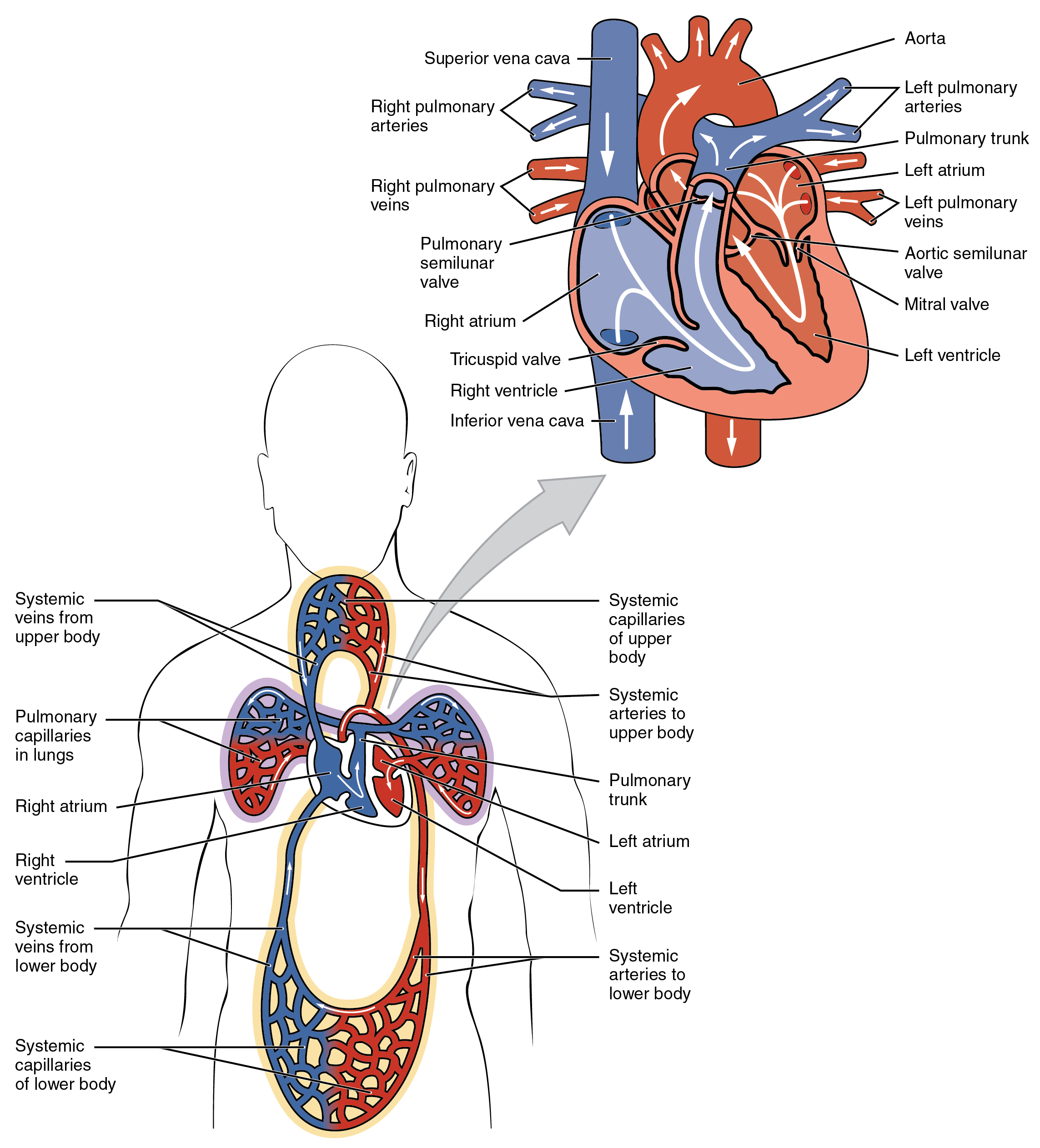
The right and left sides of the heart fill and empty simultaneously in the cardiac cycle (see below), but to understand the flow of blood through the heart, it is helpful to first follow the pathway that one molecule of blood would take through the heart as it is reoxygenated:
- Deoxygenated blood that is carrying carbon dioxide and waste products from the body tissues is returned to the right atrium via the superior vena cava and the inferior vena cava.
- From the right atrium, the deoxygenated blood moves through the tricuspid valve into the right ventricle.
- The right ventricle pumps deoxygenated blood through the pulmonary semilunar valve into the pulmonary trunk, which splits into the right and left pulmonary arteries, leading toward the lungs. These arteries branch many times before reaching the pulmonary capillaries, where gas exchange occurs: carbon dioxide exits the blood and oxygen enters. The pulmonary arteries are the only arteries in the postnatal body that carry deoxygenated blood. Did you notice that they are often coloured blue on diagrams of the heart?
- Freshly oxygenated blood returns from the lungs to the left atrium via the pulmonary veins. These veins are the only postnatal veins in the body that carry highly oxygenated blood, and are often coloured red on heart images.
- From the left atrium, the blood moves through the bicuspid (mitral) valve into the left ventricle.
- The left ventricle pumps blood through the aortic semilunar valve, into the aorta, delivering oxygenated blood to all parts of the body.
Cardiac Cycle
The period of time that begins with contraction of the atria and ends with ventricular relaxation is known as the cardiac cycle (Figure 10.17). Diastole is the portion of the cycle in which the heart is relaxed and the atria and ventricles are filling with blood. The atrioventricular (tricuspid and bicuspid) valves are open, so that blood can move from the atria to the ventricles. Systole is the portion of the cycle in which the heart contracts, the atrioventricular valves slam shut, and the ventricles eject blood to the lungs and to the body through the open semilunar valves. Once this phase ends, the semilunar valves close, in preparation for another filling phase. Both the atria and ventricles undergo systole and diastole, and it is essential that these components be carefully regulated and coordinated to ensure blood is pumped efficiently to the body.
Unless otherwise indicated, this chapter contains material adapted from chapter 22 and 21 in Anatomy, Physiology, and Medical Language by NSCC, Kimberlee Carter, Marie Rutherford, and Douglas College Biology Department as well as chapter 19 in Anatomy and Physiology (on OpenStax), by Betts, et al. and is used under a a CC BY 4.0 international license. Download and access OpenStax Anatomy and Physiology for free at https://openstax.org/books/anatomy-and-physiology-2e/