Lab 2: Cells and Tissues I
Cell Structures, Cell Cycle, Epithelial Tissue
When you are prepared for the Test on Week 2 Learning Objectives in Week 3, you will be able to:
- Identify parts of a microscope and their functions.
- Describe and demonstrate correct usage of a microscope by observing slides of cells and tissues with proper lighting and magnification.
- Identify cell structures visible at different scales and describe their functions.
- Identify stages of the cell cycle and mitosis.
- Differentiate between the general functions of epithelial, connective, muscle, and nervous tissues.
- Identify epithelial tissue types, their features, and their basic functions.
All living things are composed of cells. This remarkable fact was first discovered some 300 years ago and is one of the tenets of the Cell Theory, a basic theory of biology. Cell Theory states that:
- All life is composed of cells
- Cells are the fundamental units which possess all the characteristics of living things
- New cells can only come into existence by the division of previously existing cells
Notice that this scientific concept about life is called a theory. In science, unlike the layman’s definition, the word theory is used for a hypothesis about which there is a large body of convincing evidence. Under experimental conditions all observations have thus far confirmed the theory. The evidence that helped formulate the theory was obtained using the microscope. The microscope is of enormous importance to biology and has extended our ability to see beyond the scope of the naked eye.
The Compound Microscope
The microscope consists of a stand (base + arm), on which is mounted the stage (for holding microscope slides) and lenses. The lens that you look through is the ocular lens (paired in binocular scopes); the lens that focuses on the specimen is the objective.
Your microscope has three objective lenses of varying magnifications (4x, 10x, and 40x) mounted on a revolving nosepiece.
Positioning the specimen requires that you turn the mechanical stage controls, which operate the slide bracket on the surface of the stage. One control moves the specimen in the x-direction, and the other moves the specimen in the y-direction.
Focusing on the specimen is achieved by knobs that move the stage up and down, so that it is closer or farther from the objective lens. There are two knobs, an outer coarse focus and an inner fine focus.
The substage condenser directs light through the slide into the objective. An iris diaphragm on the substage condenser controls the amount of light reaching the objective, and also affects the contrast of the specimen.
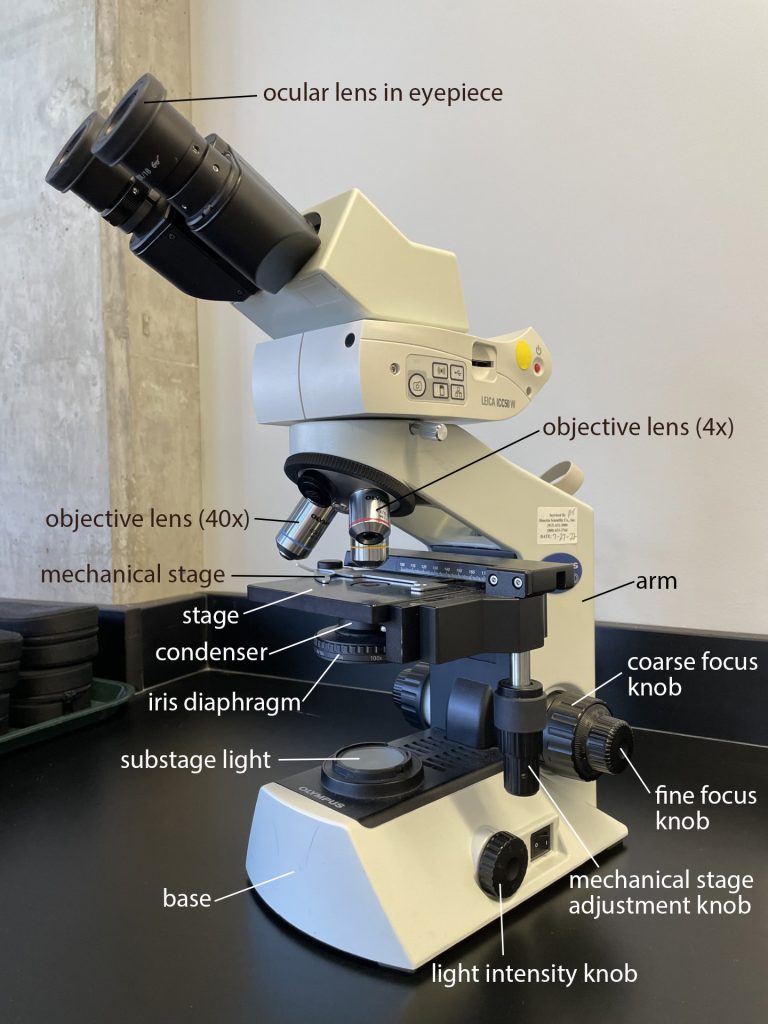
Magnification
The compound microscope has two sets of lenses; the ocular lens (or eye piece) which magnifies an object 10 times its normal size, and the objective lenses located on a revolving nosepiece. Rotate the nosepiece and notice how each objective lens clicks into place. Each objective lens has a different magnification of power written on it (such as 4, 10, or 40). This number is the power of magnification for each of the objective lenses. For total magnification multiply the ocular power (10x) times the objective lens that is in place. For example, if you have a 10x ocular and a 10x objective, the total magnification is: 10x × 10x = 100x.
Use this information to fill in the following table:
Ocular Lens | Objective Lens | Total Magnification | ||
---|---|---|---|---|
10 | × | ________ (scanning) | = | ________ |
10 | × | ________ (low power) | = | ________ |
10 | × | ________ (high power) | = | ________ |
Using the Microscope
After the instructor explains the proper carrying procedures, each student should get out a compound microscope and place it before them on the bench. The instructor will then go over the procedures for using your scope.
Complete the following procedure EVERY TIME you get your microscope out and EVERY TIME you put it away.
Getting Started
- Get your microscope out of the cabinet in the lab. Carry it with TWO HANDS.
- Before plugging in your scope, always make sure that the voltage control is at its lowest level and the light switch is off.
- Plug in the microscope and turn on the light source.
- Raise the substage condenser to its top position and open the iris diaphragm all the way.
- Turn the nosepiece so that the 4x objective is lined up with the light source.
- Place a slide on the stage and use the mechanical stage controls to move it into place.
- Turn up the light to a comfortable level.
Getting a Focused Image
- Adjust the interocular distance (distance between the oculars) by gently pressing the oculars together or pulling them apart until you see a single circular field of view.
- Look through both oculars (i.e., keep both eyes open), but concentrate on the right ocular and adjust focus until the specimen is clear in your right eye.
- Now concentrate on the left ocular and turn the diopter adjustment (the moveable ring) on the left eyepiece to adjust the focus for your left eye. You should have a sense of the image suddenly “popping out” at you, sharp and clear.
Optimizing Resolution and Contrast
Resolution is the ability to distinguish two closely spaced points on your specimen, and it is always best with the iris diaphragm wide open. Contrast is the magnitude of difference between light and dark objects, and it increases as you close the aperture of the iris diaphragm. Getting the best image, then, requires that you find the right balance. Slowly open and close the iris diaphragm to get a feeling for the effect this has on your image.
Changing Magnification
Always start with the lowest power objective (4x) to get oriented. You should use the coarse focus knob to move the stage up and down and locate an area of interest. Then you can switch to higher power to examine interesting regions more closely. To change magnification, simply rotate the nosepiece to bring one of the other objectives into the light path. When you are using the 10x or 40x objective lenses, ONLY use the fine focus knob. If you use the coarse focus knob, you might bring it up into the objective lens and break it.
Finishing Up
In this order: Turn down the illumination, turn off the power, switch back to the 4X objective lens, remove your slide, and unplug the power cord and wrap it around the back. Then return your scope to the cabinet.
Cells
You developed from a single fertilized egg cell into the complex organism containing trillions of cells that you see when you look in a mirror. During this developmental process, early, undifferentiated cells differentiate and become specialized in their structure and function. These different cell types form specialized tissues that work in concert to perform all of the functions necessary for the living organism. Cellular and developmental biologists study how the continued division of a single cell leads to such complexity and differentiation.
Consider the difference between a structural cell in the skin and a nerve cell. A structural skin cell may be shaped like a flat plate (squamous) and live only for a short time before it is shed and replaced. Packed tightly into rows and sheets, the squamous skin cells provide a protective barrier for the cells and tissues that lie beneath. A nerve cell, on the other hand, may be shaped something like a star, sending out long processes up to a meter in length and may live for the entire lifetime of the organism. With their long winding appendages, nerve cells can communicate with one another and with other types of body cells and send rapid signals that inform the organism about its environment and allow it to interact with that environment. These differences illustrate one very important theme that is consistent at all organizational levels of biology: the form of a structure is optimally suited to perform particular functions assigned to that structure. Keep this theme in mind as you tour the inside of a cell and are introduced to the various types of cells in the body.
The Cell Membrane
The cell membrane is an extremely pliable structure composed primarily of back-to-back phospholipids (a “bilayer”). Cholesterol is also present, which contributes to the fluidity of the membrane, and there are various proteins embedded within the membrane that have a variety of functions. Some cells have protrusions of the cell membrane called microvilli. Microvilli increase the surface area of cells and are found in cells lining the digestive system to increase nutrient absorption.
The Cytoplasm and Cellular Organelles
All living cells in multicellular organisms contain an internal cytoplasmic compartment, and a nucleus within the cytoplasm. Cytosol, the jelly-like substance within the cell, provides the fluid medium necessary for biochemical reactions. Eukaryotic cells, including all animal cells, also contain various cellular organelles. An organelle (“little organ”) is one of several different types of membrane-enclosed bodies in the cell, each performing a unique function. Just as the various bodily organs work together in harmony to perform all of a human’s functions, the many different cellular organelles work together to keep the cell healthy and performing all of its important functions. The organelles and cytosol, taken together, compose the cell’s cytoplasm. The nucleus is a cell’s central organelle, which contains the cell’s DNA (Figure 2.2).
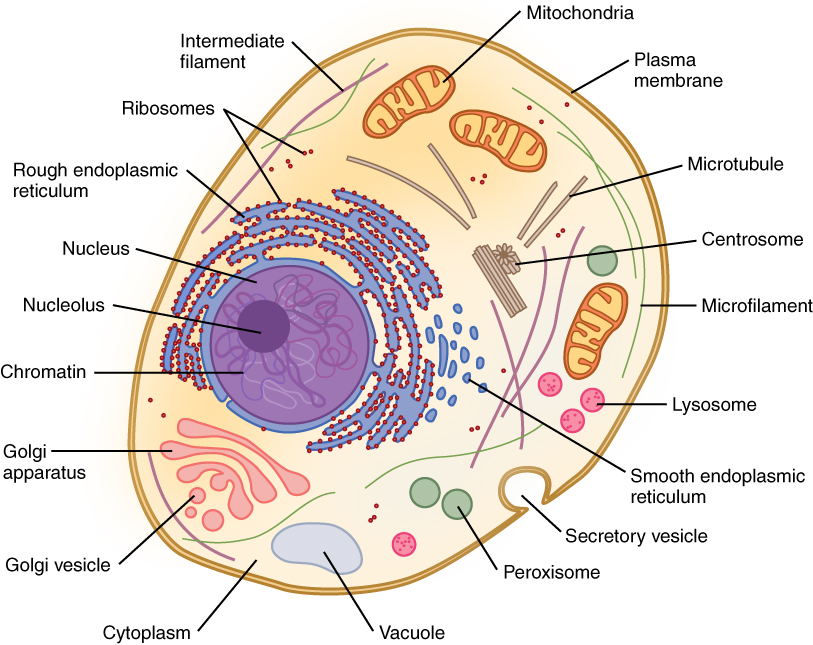
Endoplasmic Reticulum
The endoplasmic reticulum (ER) is a system of channels that is continuous with the nuclear membrane (or “envelope”) covering the nucleus and composed of the same lipid bilayer material (Figure 2.3). The ER can be thought of as a series of winding thoroughfares similar to the waterway canals in Venice. The ER has a large membranous surface and provides passages throughout much of the cell that function in transporting, synthesizing, and storing materials.
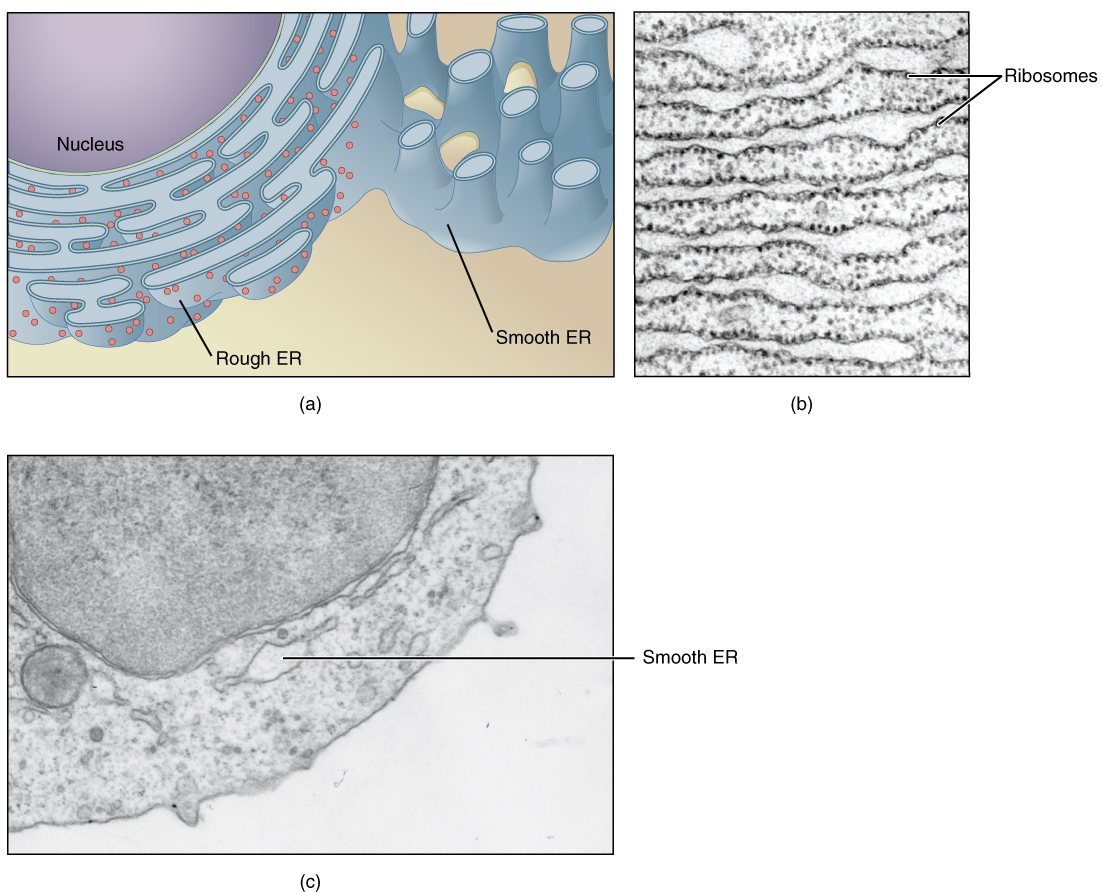
Endoplasmic reticulum can exist in two forms: rough ER and smooth ER. These two types of ER perform some very different functions and can be found in very different amounts depending on the type of cell. Rough endoplasmic reticulum is so-called because its membrane is dotted with embedded granules—organelles called ribosomes, giving the rough ER a bumpy appearance. A ribosome is an organelle that serves as the site of protein synthesis. The primary job of the rough ER is the synthesis and modification of proteins destined for the cell membrane or for export from the cell. Typically, a protein is synthesized within the ribosome and released inside the channel of the rough ER, where it is folded and modified before it is transported within a vesicle to the next stage in the packaging and shipping process: the Golgi apparatus.
The smooth endoplasmic reticulum lacks ribsomes, giving it a smooth appearance. One of the main functions of the smooth ER is in the synthesis of lipids, such as phospholipids, the main component of biological membranes, as well as steroid hormones. For this reason, cells that produce large quantities of such hormones, such as those of the female ovaries and male testes, contain large amounts of smooth ER. In addition to lipid synthesis, the smooth ER also sequesters (i.e., stores) and regulates the concentration of cellular Ca++, a function extremely important in cells of the nervous and muscular systems. The smooth ER additionally metabolizes some carbohydrates and performs a detoxification role, breaking down certain toxins.
The Golgi Apparatus
The Golgi apparatus is responsible for sorting, modifying, and shipping off the products that come from the rough ER, much like a post-office. The Golgi apparatus looks like stacked flattened discs, almost like stacks of oddly shaped pancakes. Like the ER, these discs are membranous. The Golgi apparatus has two distinct sides, each with a different role. One side of the apparatus receives products in vesicles. These products are sorted through the apparatus, and then they are released from the opposite side after being repackaged into new vesicles. If the product is to be exported from the cell, the vesicle migrates to the cell surface and fuses to the cell membrane, and the cargo is secreted (Figure 2.4).
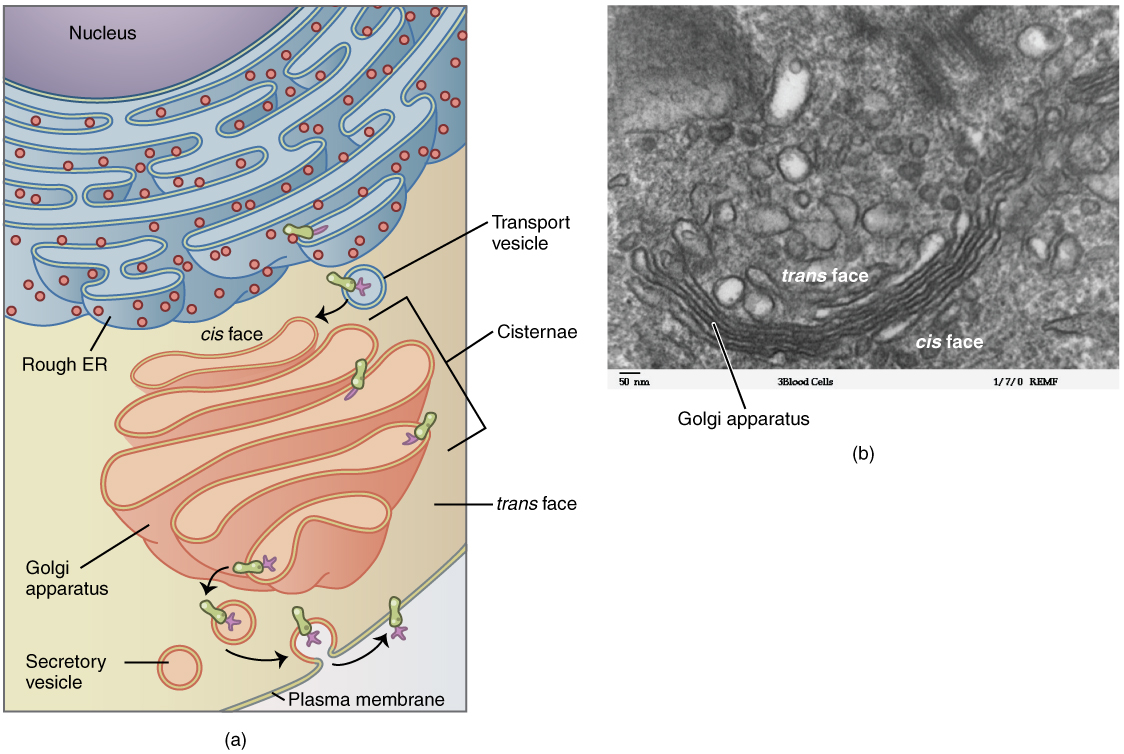
Lysosomes
Some of the protein products packaged by the Golgi include digestive enzymes that are meant to remain inside the cell for use in breaking down certain materials. The enzyme-containing vesicles released by the Golgi may form new lysosomes, or fuse with existing, lysosomes. A lysosome is an organelle that contains enzymes that can break down and digest unneeded cellular components, such as a damaged organelle, or foreign material, such as bacteria. Under certain circumstances, like in the case of damaged or unhealthy cells, lysosomes can be triggered to open up and release their digestive enzymes into the cytoplasm of the cell, killing the cell.
Mitochondria
A mitochondrion (plural = mitochondria) is a membranous, bean-shaped organelle that is the “energy transformer” of the cell. Mitochondria consist of an outer lipid bilayer membrane as well as an additional inner lipid bilayer membrane (Figure 2.5), along which a series of proteins, enzymes, and other molecules perform the biochemical reactions of cellular respiration. These reactions convert energy stored in nutrient molecules (such as glucose) into adenosine triphosphate (ATP), which provides usable cellular energy to the cell. Cells use ATP constantly, and so the mitochondria are constantly at work. Oxygen molecules are required during cellular respiration, which is why you must constantly breathe it in. One of the organ systems in the body that uses huge amounts of ATP is the muscular system because ATP is required to sustain muscle contraction. As a result, muscle cells are packed full of mitochondria. Nerve cells also need large quantities of ATP to run their sodium-potassium pumps. Therefore, an individual neuron will be loaded with over a thousand mitochondria. On the other hand, a bone cell, which is not nearly as metabolically-active, might only have a couple hundred mitochondria.
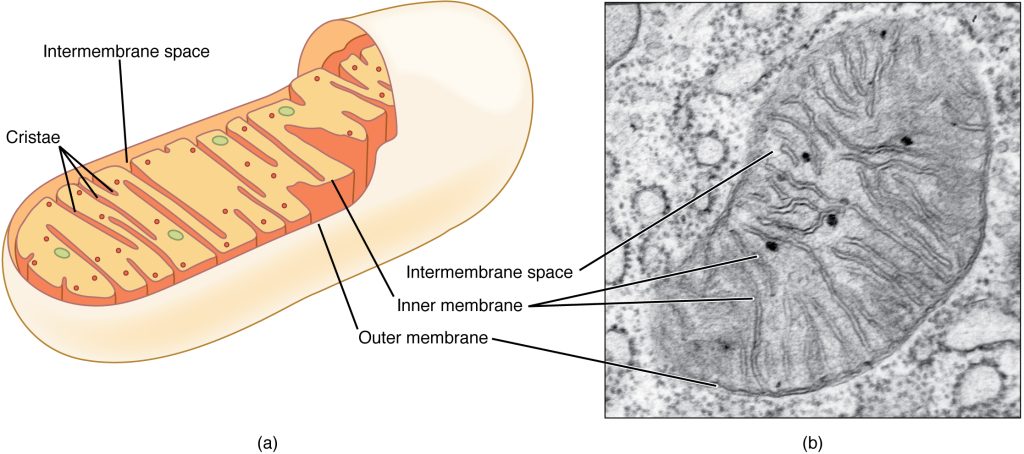
Peroxisomes
Like lysosomes, a peroxisome is a membrane-bound cellular organelle that contains mostly enzymes. Peroxisomes perform a couple of different functions, including lipid metabolism and chemical detoxification. In contrast to the digestive enzymes found in lysosomes, the enzymes within peroxisomes serve to transfer hydrogen atoms from various molecules to oxygen, producing hydrogen peroxide (H2O2). In this way, peroxisomes neutralize poisons such as alcohol. H2O2 would be toxic to the cell, but peroxisomes contain enzymes that convert H2O2 into water and oxygen. These byproducts are then safely released into the cytoplasm. Like miniature sewage treatment plants, peroxisomes neutralize harmful toxins so that they do not wreak havoc in the cells. The liver is the organ primarily responsible for detoxifying the blood before it travels throughout the body, and liver cells contain an exceptionally high number of peroxisomes.
The Cytoskeleton
The cytoskeleton is a group of fibrous proteins that play important roles in structural support for cells, cell motility, cell reproduction, and transportation of substances within the cell. The cytoskeleton forms a complex thread-like network throughout the cell consisting of three different kinds of protein-based filaments: microfilaments, intermediate filaments, and microtubules (Figure 2.6). Microtubules are the thickest of the three and maintain cell shape and structure, help resist compression of the cell, and play a role in positioning the organelles within the cell. Microtubules also make up two types of cellular appendages important for motion: cilia and flagella. Cilia are found on many cells of the body, including the epithelial cells that line the airways of the respiratory system. Cilia beat constantly, moving waste materials such as dust, mucus, and bacteria upward through the airways, away from the lungs and toward the mouth. A flagellum (plural = flagella) is an appendage larger than a cilium and specialized for cell locomotion. The only flagellated cell in humans is the sperm cell that must propel itself towards female egg cells.
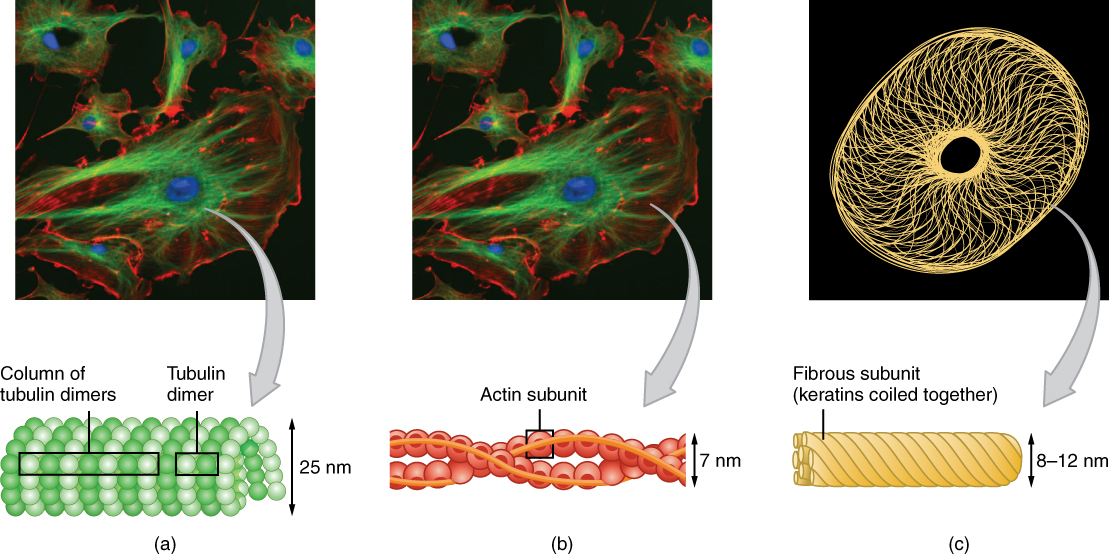
A very important function of microtubules is to set the paths (somewhat like railroad tracks) along which the genetic material can be pulled (a process requiring ATP) during cell division, so that each new daughter cell receives the appropriate set of chromosomes. A centrosome is found near the nucleus of cells and consists of two short, identical microtubule structures called centrioles. A centriole can serve as the cellular origin point for microtubules extending outward or can assist with the separation of DNA during cell division.
In contrast with microtubules, the microfilament is a thinner type of cytoskeletal filament. Actin, a protein that forms chains, is the primary component of these microfilaments. Actin fibers, twisted chains of actin filaments, constitute a large component of muscle tissue and, along with the protein myosin, are responsible for muscle contraction. Like microtubules, actin filaments are long chains of single subunits (called actin subunits). In muscle cells, these long actin strands, called thin filaments, are “pulled” by thick filaments of the myosin protein to contract the cell.
The final cytoskeletal filament is the intermediate filament. As its name would suggest, an intermediate filament is a filament intermediate in thickness between the microtubules and microfilaments. Intermediate filaments, in concert with the microtubules, are important for maintaining cell shape and structure.
The Nucleus
The nucleus is the largest and most prominent of a cell’s organelles (Figure 2.7). The nucleus is generally considered the control center of the cell because it stores all of the genetic instructions for manufacturing proteins. Inside the nucleus lies the blueprint that dictates everything a cell will do and all of the products it will make. This information is stored within DNA. The nucleus sends “commands” to the cell via molecular messengers that translate the information from DNA. Each cell in your body (with the exception of germ cells) contains the complete set of your DNA. When a cell divides, the DNA must be duplicated so that the each new cell receives a full complement of DNA. The following section will explore the structure of the nucleus and its contents, as well as the process of DNA replication.
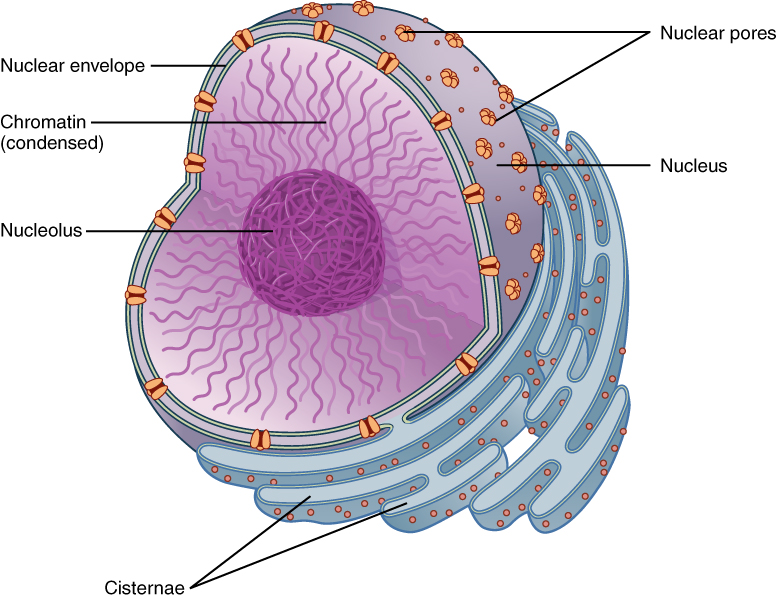
Organization of the Nucleus and Its DNA
Like most other cellular organelles, the nucleus is surrounded by a membrane called the nuclear envelope. This membranous covering consists of two adjacent lipid bilayers with a thin fluid space in between them. Spanning these two bilayers are nuclear pores. A nuclear pore is a tiny passageway for the passage of proteins, RNA, and solutes between the nucleus and the cytoplasm. Proteins called pore complexes lining the nuclear pores regulate the passage of materials into and out of the nucleus.
Inside the nuclear envelope is a gel-like nucleoplasm with solutes that include the building blocks of nucleic acids. There also can be a dark-staining mass often visible under a simple light microscope, called a nucleolus (plural = nucleoli). The nucleolus is a region of the nucleus that is responsible for manufacturing the RNA necessary for construction of ribosomes. Once synthesized, newly made ribosomal subunits exit the cell’s nucleus through the nuclear pores.
The genetic instructions that are used to build and maintain an organism are arranged in an orderly manner in strands of DNA. Within the nucleus are threads of chromatin composed of DNA and associated proteins. When a cell is in the process of division, the chromatin condenses into chromosomes, so that the DNA can be safely transported to the “daughter cells.” The chromosome is composed of DNA and proteins; it is the condensed form of chromatin. It is estimated that humans have almost 22,000 genes distributed on 46 chromosomes.
The Cell Cycle and Cellular Division
Cells in the body replace themselves over the lifetime of a person. For example, the cells lining the gastrointestinal tract must be frequently replaced when constantly “worn off” by the movement of food through the gut. While there are a few cells in the body that do not undergo cell division (such as gametes, red blood cells, most neurons, and some muscle cells), most somatic cells divide regularly. A somatic cell is a general term for a body cell, and all human cells, except for the cells that produce eggs and sperm (which are referred to as germ cells), are somatic cells. Somatic cells contain two copies of each of their chromosomes (one copy received from each parent). A homologous pair of chromosomes is the two copies of a single chromosome found in each somatic cell. The human is a diploid organism, having 23 homologous pairs of chromosomes in each of the somatic cells.
The cell cycle is the sequence of events in the life of the cell from the moment it is created at the end of a previous cycle of cell division until it then divides itself, generating two new cells. One “turn” or cycle of the cell cycle consists of two general phases: interphase, followed by mitosis and cytokinesis (Figure 2.8). Interphase is the period of the cell cycle during which the cell is not dividing. The majority of cells are in interphase most of the time. During interphase, the cell grows and carries out all normal metabolic functions, as well as prepares to divide by replicating its DNA. After DNA replication but before cell division, each cell actually contains two copies of each chromosome. Each copy of the chromosome is referred to as a sister chromatid and is physically bound to the other copy. The centromere is the structure that attaches one sister chromatid to another.
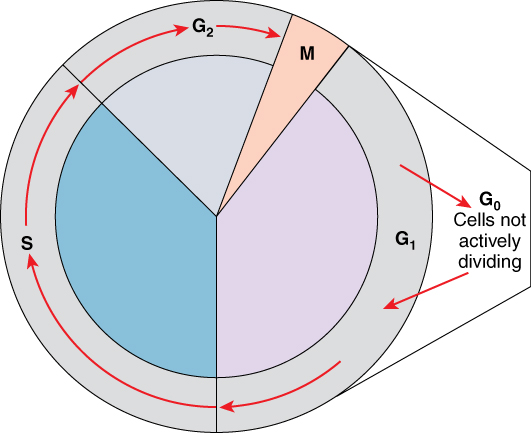
Mitosis is the division of genetic material, during which the cell nucleus breaks down and two new, fully functional, nuclei are formed. Cytokinesis divides the cytoplasm into two distinctive cells. The mitotic phase of the cell typically takes between 1 and 2 hours. During this phase, a cell undergoes two major processes. First, it completes mitosis, during which the contents of the nucleus are equitably pulled apart and distributed between its two halves. Cytokinesis then occurs, dividing the cytoplasm and cell body into two new cells. Mitosis is divided into four major stages that take place after interphase (Figure 2.9) and in the following order: prophase, metaphase, anaphase, and telophase.
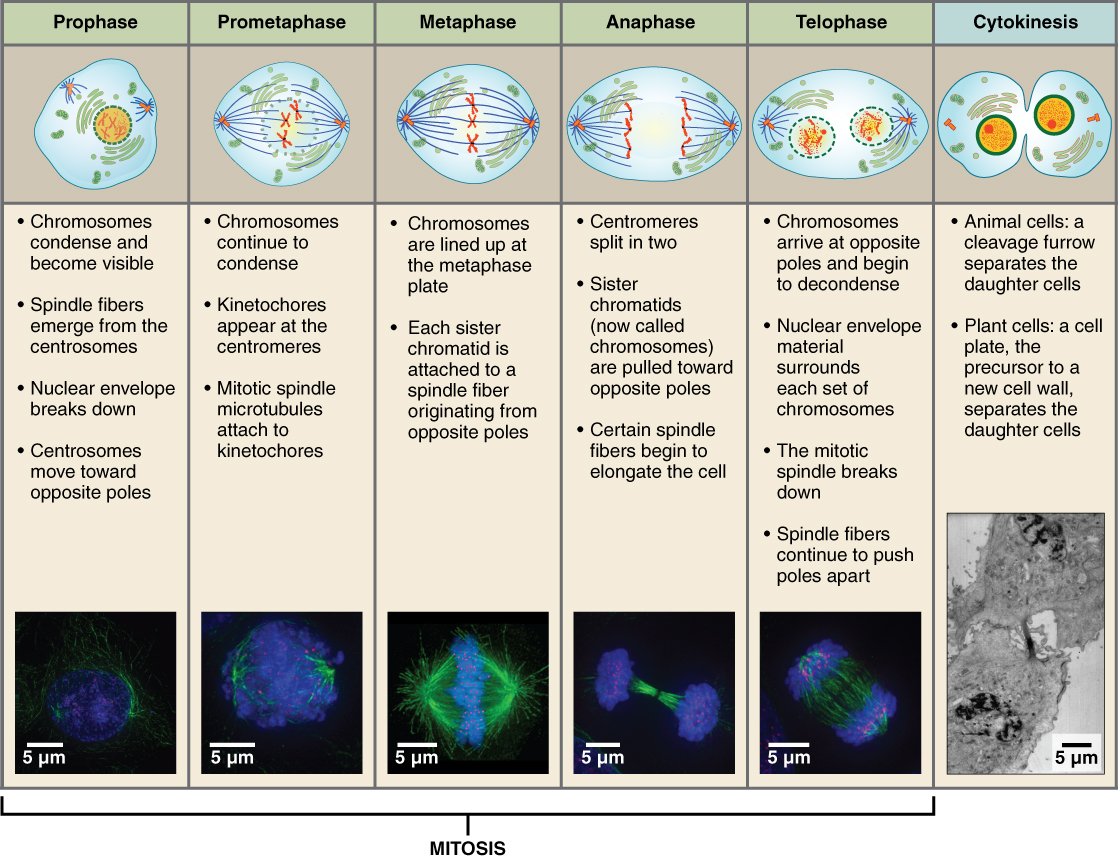
Prophase is the first phase of mitosis, during which the loosely packed chromatin coils and condenses into visible chromosomes. During prophase, each chromosome becomes visible with its identical partner attached, forming the familiar X-shape of sister chromatids. The nucleolus disappears early during this phase, and the nuclear envelope also disintegrates. Also during prophase, the cell contains two centrosomes side-by-side, which begin to move apart. As the centrosomes migrate to two different sides of the cell, microtubules begin to extend from each like long fingers from two hands extending toward each other. The mitotic spindle is the structure composed of the centrosomes and their emerging microtubules.
Near the end of prophase there is an invasion of the nuclear area by microtubules from the mitotic spindle. The nuclear membrane has disintegrated, and the microtubules attach themselves to the centromeres that adjoin pairs of sister chromatids. This stage is referred to as late prophase or “prometaphase” to indicate the transition between prophase and metaphase.
Metaphase is the second stage of mitosis. During this stage, the sister chromatids, with their attached microtubules, line up along a linear plane in the middle of the cell and mitotic spindle. This plane is called the metaphase plate, and it is formed between the centrosomes that are now located at either end of the cell.T he microtubules are now poised to pull apart the sister chromatids and bring one from each pair to each side of the cell.
Anaphase is the third stage of mitosis. Anaphase takes place over a few minutes, when the pairs of sister chromatids are separated from one another, forming individual chromosomes once again. These chromosomes are pulled to opposite ends of the cell as the microtubules shorten. Each end of the cell receives one partner from each pair of sister chromatids, ensuring that the two new daughter cells will contain identical genetic material.
Telophase is the final stage of mitosis. Telophase is characterized by the formation of two new daughter nuclei at either end of the dividing cell. These newly formed nuclei surround the genetic material, which uncoils such that the chromosomes return to loosely packed chromatin. Nucleoli also reappear within the new nuclei, and the mitotic spindle breaks apart, each new cell receiving its own complement of DNA, organelles, membranes, and centrioles. At this point, the cell is already beginning to split in half as cytokinesis begins.
During cytokinesis, a contractile band called the cleavage furrow forms around the midline of the cell. This contractile band squeezes the two cells apart until they finally separate. Two new cells are now formed.
Tissues
The body contains at least 200 distinct cell types. These cells contain essentially the same internal structures, yet they vary enormously in shape and function. The different types of cells are not randomly distributed throughout the body; rather they occur in organized layers, a level of organization referred to as tissue.
The term tissue is used to describe a group of cells found together in the body. The cells within a tissue share a common embryonic origin. Microscopic observation reveals that the cells in a tissue share morphological features and are arranged in an orderly pattern that achieves the tissue’s functions. From the evolutionary perspective, tissues appear in more complex organisms. For example, multicellular protists, ancient eukaryotes, do not have cells organized into tissues. Having tissue level organization increases the efficiency of the body as different shapes and internal structures are better suited to carry out different functions. Having different tissues for different functions allows for a greater speed of activity and a greater effectiveness in performing the various activities.
Although there are many types of cells in the human body, they are organized into four broad categories of tissues: epithelial, connective, muscle, and nervous (Figure 2.10). Each of these categories is characterized by specific functions that contribute to the overall health and maintenance of the body. Epithelial tissue, also referred to as epithelium, refers to the sheets of cells that cover exterior surfaces of the body, lines internal cavities and passageways, and forms certain glands. Connective tissue, as its name implies, binds the cells and organs of the body together and functions in the protection, support, and integration of all parts of the body. Muscle tissue is excitable, responding to stimulation and contracting to provide movement, and occurs as three major types: skeletal muscle, smooth muscle, and cardiac muscle in the heart. Nervous tissue is also excitable, allowing the propagation of electrochemical signals in the form of nerve impulses that communicate between different regions of the body.
The next level of organization is the organ, where several types of tissues come together to form a working unit. Just as knowing the structure and function of cells helps you in your study of tissues, knowledge of tissues will help you understand how organs function.
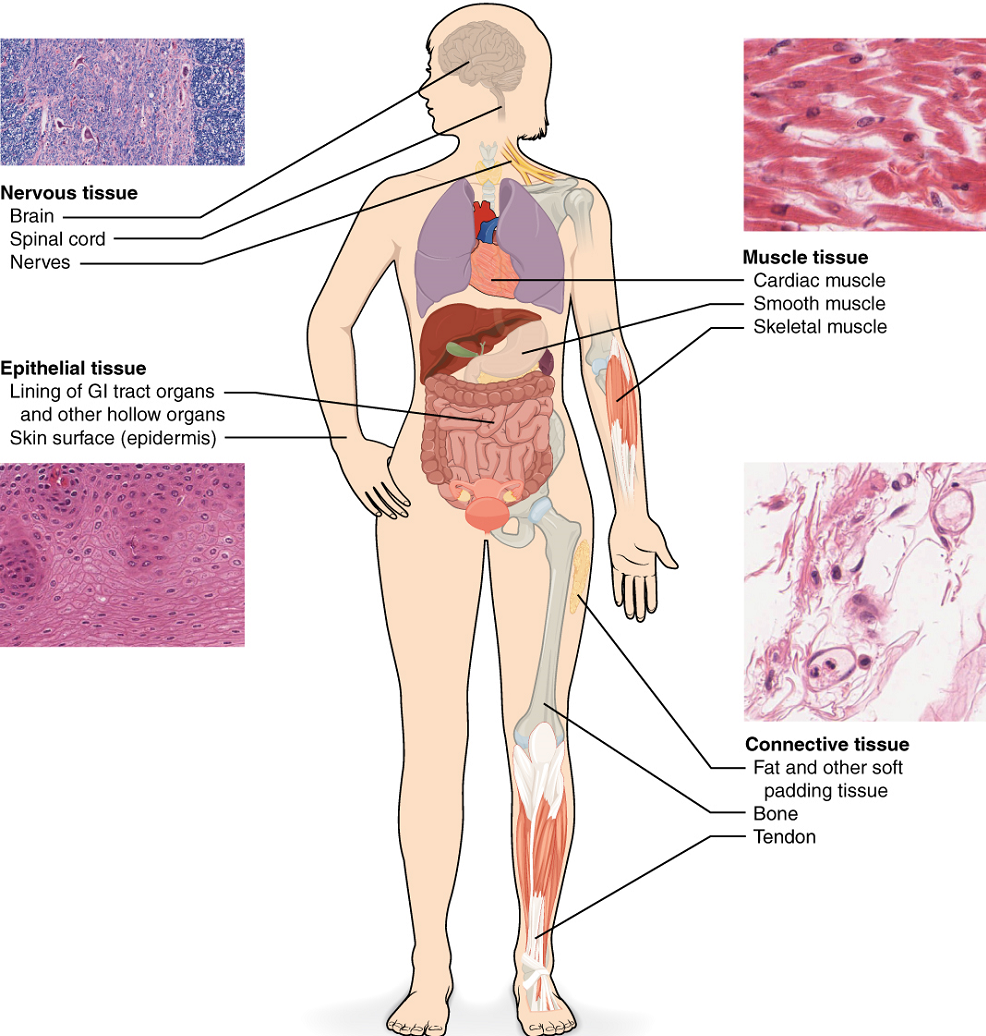
This week we will just examine epithelial tissue. Next week we will look more closely at the remaining three tissue types.
Epithelial Tissue
Most epithelial tissues are essentially large sheets of cells covering all the surfaces of the body exposed to the outside world, and lining the outside of organs and the body cavities. Epithelium also forms much of the glandular tissue of the body. Skin is not the only area of the body exposed to the outside. Other areas include the airways, the digestive tract, as well as the urinary and reproductive systems, all of which are lined by an epithelium. Hollow organs and body cavities that do not connect to the exterior of the body, which includes, blood vessels and serous membranes, are lined by endothelium (plural = endothelia), which is a type of epithelium.
Epithelial tissues provide the body’s first line of protection from physical, chemical, and biological wear and tear. The cells of an epithelium act as gatekeepers of the body controlling permeability and allowing selective transfer of materials across a physical barrier. All substances that enter the body must cross an epithelium. Many epithelial cells are capable of secretion and release mucous and specific chemical compounds onto their apical surfaces. The epithelium of the small intestine releases digestive enzymes, for example. Cells lining the respiratory tract secrete mucous that traps incoming microorganisms and particles.
General Structure of Epithelial Tissue
All epithelia share some important structural and functional features. This tissue is highly cellular, with little or no extracellular material present between cells. The epithelial cells exhibit polarity with differences in structure and function between the exposed or apical facing surface of the cell and the basal surface close to the underlying body structures. Particular structures found in some epithelial cells are an adaptation to specific functions. Certain organelles are segregated to the basal sides, whereas other organelles and extensions, such as cilia, when present, are on the apical surface. The basal lamina of the epithelial cells attaches to underlying connective tissue. via a basement membrane that helps hold it all together.
Epithelial tissues are nearly completely avascular. For instance, no blood vessels cross the basement membrane to enter the tissue, and nutrients must come by diffusion or absorption from underlying tissues or the surface. Many epithelial tissues are capable of rapidly replacing damaged and dead cells. Sloughing off of damaged or dead cells is a characteristic of surface epithelium and allows our airways and digestive tracts to rapidly replace damaged cells with new cells.
Classification of Epithelial Tissues
Epithelial tissues are classified according to the shape of the cells and number of the cell layers formed (Figure 2.11). Cell shapes can be squamous (flattened and thin), cuboidal (boxy, as wide as it is tall), or columnar (rectangular, taller than it is wide). Similarly, the number of cell layers in the tissue can be one—where every cell rests on the basal lamina—which is a simple epithelium, or more than one, which is a stratified epithelium and only the basal layer of cells rests on the basal lamina. Pseudostratified (pseudo- = “false”) describes tissue with a single layer of irregularly shaped cells that give the appearance of more than one layer. Transitional describes a form of specialized stratified epithelium in which the shape of the cells can vary.
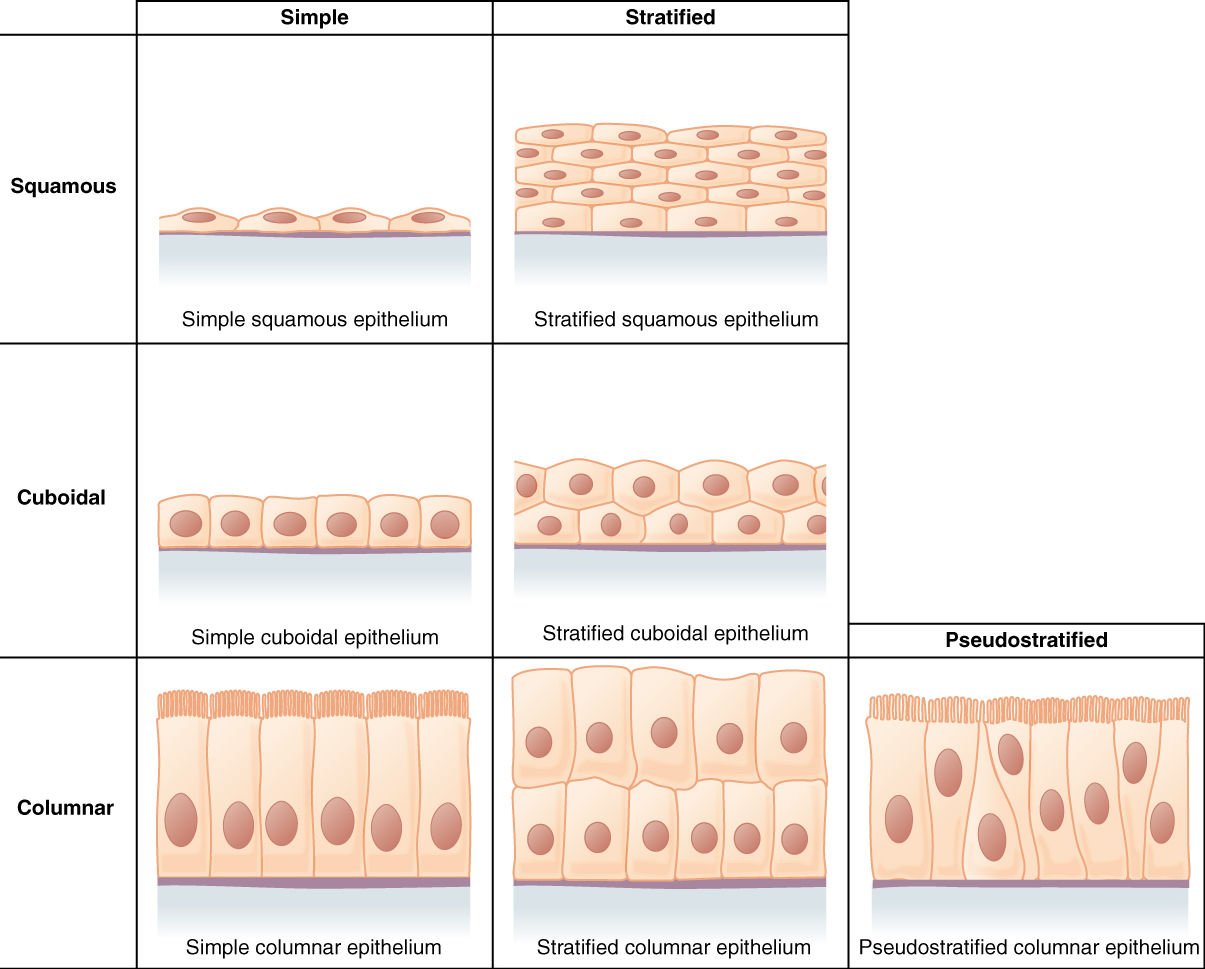
Simple Epithelium
The shape of the cells in the single cell layer of simple epithelium reflects the functioning of those cells (Figure 2.12). The cells in simple squamous epithelium have the appearance of thin scales. Squamous cell nuclei tend to be flat, horizontal, and elliptical, mirroring the form of the cell. Simple squamous epithelium, because of the thinness of the cell, is present where rapid passage of chemical compounds is observed. The alveoli of lungs where gases diffuse, segments of kidney tubules, and the lining of capillaries are also made of simple squamous epithelial tissue.
In simple cuboidal epithelium, the nucleus of the box-like cells appears round and is generally located near the center of the cell. These epithelia are active in the secretion and absorptions of molecules. Simple cuboidal epithelia are observed in the lining of the kidney tubules and in the ducts of glands.
In simple columnar epithelium, the nucleus of the tall column-like cells tends to be elongated and located in the basal end of the cells. Like the cuboidal epithelia, this epithelium is active in the absorption and secretion of molecules. Simple columnar epithelium forms the lining of some sections of the digestive system and parts of the female reproductive tract. Ciliated columnar epithelium is composed of simple columnar epithelial cells with cilia on their apical surfaces. These epithelial cells are found in the lining of the uterine tubes and parts of the respiratory system, where the beating of the cilia helps remove particulate matter.
Pseudostratified columnar epithelium is a type of epithelium that appears to be stratified but instead consists of a single layer of irregularly shaped and differently sized columnar cells. In pseudostratified epithelium, nuclei of neighbouring cells appear at different levels rather than clustered in the basal end. The arrangement gives the appearance of stratification; but in fact, all the cells are in contact with the basal lamina, although some do not reach the apical surface. Pseudostratified columnar epithelium is found in the respiratory tract, where some of these cells have cilia.
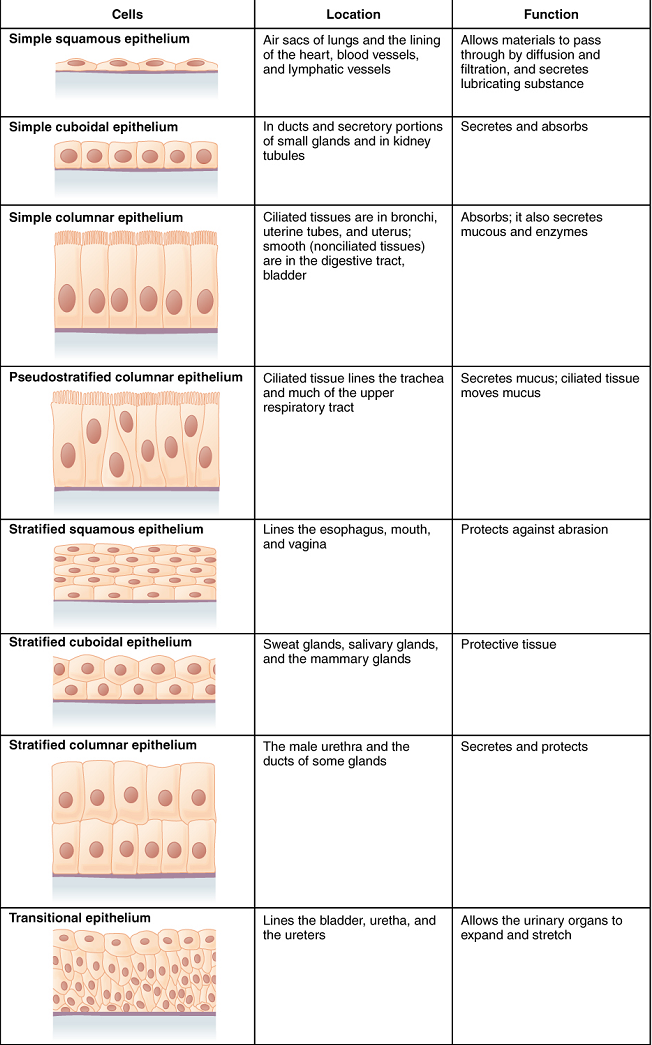
Stratified Epithelium
A stratified epithelium consists of several stacked layers of cells. This epithelium protects against physical and chemical wear and tear. The stratified epithelium is named by the shape of the most apical layer of cells, closest to the free space (Figure 2.12).
Stratified squamous epithelium is the most common type of stratified epithelium in the human body. The apical cells are squamous, whereas the basal layer contains either columnar or cuboidal cells. The top layer may be covered with dead cells filled with keratin. Mammalian skin is an example of this keratinized stratified squamous epithelium. The lining of the mouth cavity is an example of an nonkeratinized stratified squamous epithelium. Stratified cuboidal epithelium and stratified columnar epithelium can also be found in certain glands and ducts, but are uncommon in the human body.
Transitional epithelium is called that because of the gradual changes in the shapes of the apical cells as the bladder fills with urine. It is found only in the urinary system, specifically the ureters and urinary bladder. When the bladder is empty, this epithelium is convoluted and has cuboidal apical cells with convex, umbrella shaped, apical surfaces. As the bladder fills with urine, this epithelium loses its convolutions and the apical cells transition from cuboidal to squamous. It appears thicker and more multi-layered when the bladder is empty, and more stretched out and less stratified when the bladder is full and distended.
Unless otherwise indicated, this chapter contains material adapted from Biology I Laboratory Manual from Lumen Learning, Anatomy, Physiology, and Medical Language by NSCC, Kimberlee Carter, Marie Rutherford, and Douglas College Biology Department, and Anatomy and Physiology (on OpenStax), by Betts, et al. and is used under a a CC BY 4.0 international license. Download and access OpenStax Anatomy and Physiology for free at https://openstax.org/books/anatomy-and-physiology/pages/1-introduction.