Lab 8: Nervous system I – Nervous Tissue, Brain, Spinal Cord, and Cranial Nerves
Nervous tissue, structures of the central and peripheral nervous systems
Learning Objectives
When you are prepared for the Test on Week 8 Learning Objectives in Week 9, you will be able to:
- Identify structures of the neuron and describe their functions.
- Identify regions of the brain and describe their functions.
- Identify cerebral lobes and functional areas of the brain.
- Identify meninges of the central nervous system and their functions.
- Describe where cerebrospinal fluid is created, circulates, and is recycled.
- Identify cranial nerves, describing their basic function and whether each is sensory, motor, or both.
- Identify structures of the spinal cord region and their functions.
The nervous system is a complex organ system that sends signals throughout the body to control both voluntary and involuntary functions. The nervous system can detect many different forms of stimuli, integrate those stimuli, and respond to them by sending signals out to the muscle cells or glands. This week we will look at the cells and tissue of the nervous system capable of sending signals and the organs where those signals can be processed and integrated. Next week we will look at the structures capable of detecting stimuli, when we look at the special senses.
Divisions of the Nervous System
The nervous system can be divided into two major regions: the central and peripheral nervous systems. The central nervous system (CNS) is the brain and spinal cord, and the peripheral nervous system (PNS) is everything else (Figures 8.1 and 8.2). The brain is contained within the cranial cavity of the skull, and the spinal cord is contained within the vertebral cavity of the vertebral column. It is a bit of an oversimplification to say that the CNS is what is inside these two cavities and the peripheral nervous system is outside of them, but that is one way to start to think about it. In actuality, there are some elements of the peripheral nervous system that are within the cranial or vertebral cavities. The peripheral nervous system is so named because it is on the periphery—meaning beyond the brain and spinal cord. Depending on different aspects of the nervous system, the dividing line between central and peripheral is not necessarily universal.
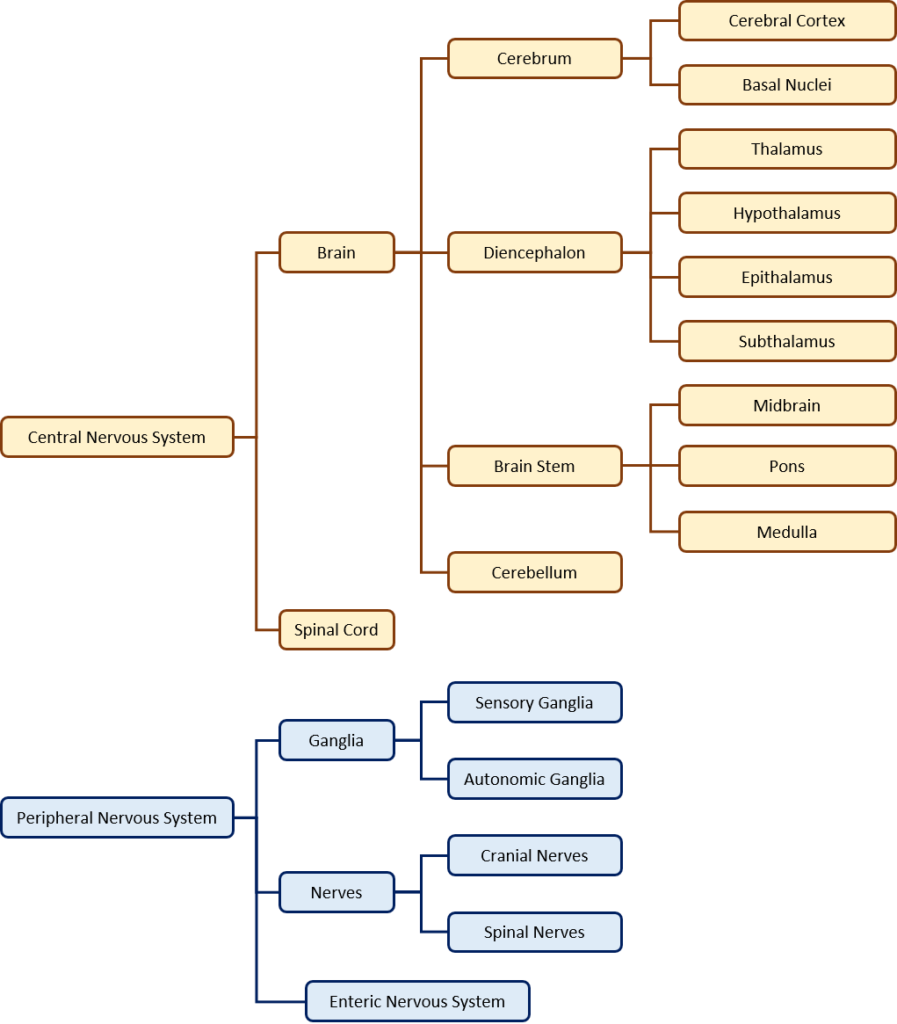
The nervous system can also be organized into functional divisions, based on sensing, integrating, and responding to stimuli (Figure 8.3). The response division is sometimes called the motor division, because it often involves signaling muscle tissue.
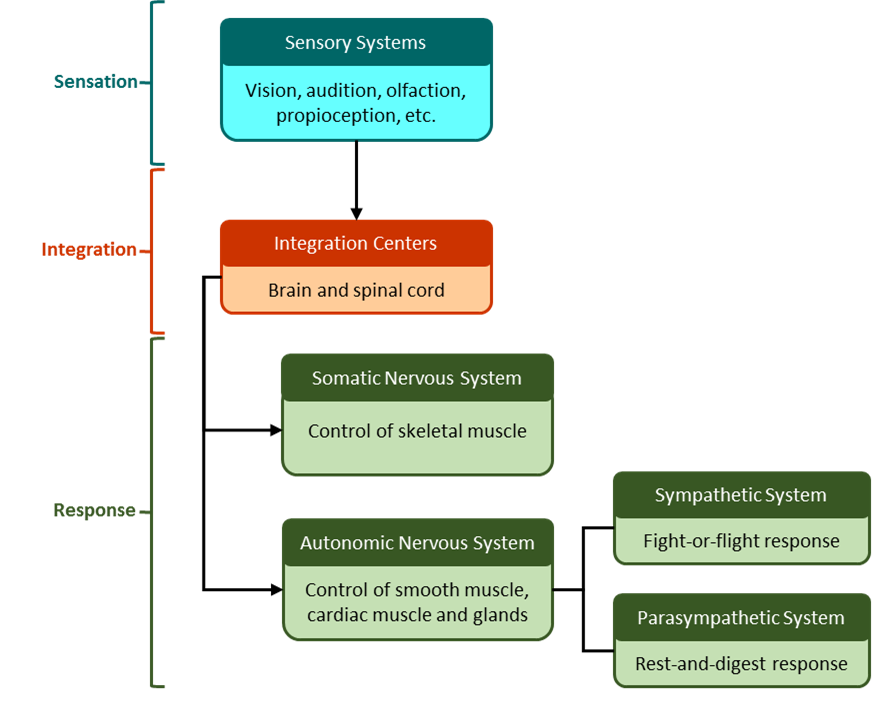
Divisions of the nervous system can also be functionally classified as somatic and autonomic. The somatic nervous system (SNS) is responsible for conscious perception and voluntary motor responses. Voluntary motor response means the contraction of skeletal muscle, but those contractions are not always voluntary in the sense that you have to want to perform them. Some somatic motor responses are reflexes, and often happen without a conscious decision to perform them. If your friend jumps out from behind a corner and yells “Boo!” you will be startled and you might scream or leap back. You didn’t decide to do that, and you may not have wanted to give your friend a reason to laugh at your expense, but it is a reflex involving skeletal muscle contractions. Other motor responses become automatic (in other words, unconscious) as a person learns motor skills (referred to as “habit learning” or “procedural memory”).
The autonomic nervous system (ANS) is responsible for involuntary control of the body, usually for the sake of homeostasis (regulation of the internal environment). Sensory input for autonomic functions can be from sensory structures tuned to external or internal environmental stimuli. The motor output extends to smooth and cardiac muscle as well as glandular tissue. The role of the autonomic system is to regulate the organ systems of the body, which usually means to control homeostasis. Sweat glands, for example, are controlled by the autonomic system. When you are hot, sweating helps cool your body down. That is a homeostatic mechanism. But when you are nervous, you might start sweating also. That is not homeostatic, it is the physiological response to an emotional state.
There is another division of the nervous system that describes functional responses. The enteric nervous system (ENS) is responsible for controlling the smooth muscle and glandular tissue in your digestive system. It is a large part of the PNS, and is not dependent on the CNS. It is sometimes valid, however, to consider the enteric system to be a part of the autonomic system because the neural structures that make up the enteric system are a component of the autonomic output that regulates digestion. There are some differences between the two, but for our purposes here there will be a good bit of overlap. See Figure 8.4 for examples of where these divisions of the nervous system can be found.
Nervous Tissue
Nervous tissue, present in both the CNS and PNS, contains two basic types of cells: neurons and glial cells. A glial cell is one of a variety of cells that provide a framework of tissue that supports the neurons and their activities. The neuron is the more functionally important of the two, in terms of the communicative function of the nervous system. Neurons are responsible for the electrical signals that communicate information about sensations, and that produce movements in response to those stimuli, along with inducing thought processes within the brain. An important part of the function of neurons is in their structure, or shape. The three-dimensional shape of these cells makes the immense numbers of connections within the nervous system possible.
Neurons are cells and therefore have a soma, or cell body, which contains the nucleus and most of the major organelles. But what makes neurons special is that they have many extensions of their cell membranes, which are generally referred to as processes. Neurons are usually described as having one, and only one, axon—a fiber that emerges from the cell body and projects to target cells. That single axon can branch repeatedly to communicate with many target cells. It is the axon that propagates the nerve impulse, which is communicated to one or more cells. The other processes of the neuron are dendrites, which receive information from other neurons at specialized areas of contact called synapses. The dendrites are usually highly branched processes, providing locations for other neurons to communicate with the cell body. Information flows through a neuron from the dendrites, across the cell body, and down the axon. This gives the neuron a polarity—meaning that information flows in this one direction. Figure 8.5 shows the relationship of these parts to one another.
Where the axon emerges from the cell body, there is a special region referred to as the axon hillock. This is a tapering of the cell body toward the axon fiber. Many axons are wrapped by an insulating substance called myelin. Myelin acts as insulation much like the plastic or rubber that is used to insulate electrical wires. A key difference between myelin and the insulation on a wire is that there are gaps in the myelin covering of an axon. Each gap is called a neurofibril node (=node of Ranvier) and is important to the way that electrical signals travel down the axon. The length of the axon between each gap, which is wrapped in myelin, is referred to as an axon segment. At the end of the axon is the axon terminal, where there are usually several branches extending toward the target cell, each of which ends in an enlargement called a synaptic end bulb. These bulbs are what make the connection with the target cell at the synapse.
The myelin sheath is made from glial cells, called oligodendrocytes in the central nervous system, or neurolemmocytes (=Schwann cells) in the peripheral nervous system. One oligodendrocyte will provide the myelin for multiple axon segments, either for the same axon or for separate axons. Schwann cells are different than oligodendrocytes, in that a Schwann cell wraps around a portion of only one axon segment and no others. The neurolemmocyte is wrapped around the axon several times with little to no cytoplasm between the glial cell layers. The nucleus and cytoplasm of the Schwann cell are on the edge of the myelin sheath (Figure 8.6).
Signals travel down the axon of a neuron in the form of action potentials, which are localized changes in membrane potential, one triggering the next, until the signal reaches the axon terminal. Action potentials can propagate much more quickly down a myelinated axon than an unmyelinated axon because the action potential basically jumps from one node to the next. This is called saltatory conduction (saltare = “to leap”).
White and Gray matter
Looking at nervous tissue, there are regions that predominantly contain cell bodies and regions that are largely composed of just myelinated axons. These two regions within nervous system structures are often referred to as gray matter (the regions with many cell bodies and dendrites) or white matter (the regions with many axons). Figure 8.7 demonstrates the appearance of these regions in the brain and spinal cord. The colors ascribed to these regions are what would be seen in “fresh,” or unstained, nervous tissue. Gray matter is not necessarily gray. It can be pinkish because of blood content, or even slightly tan, depending on how long the tissue has been preserved. But white matter is white because axons are insulated by the lipid-rich myelin sheath. Lipids can appear as white (“fatty”) material, much like the fat on a raw piece of chicken or beef.
The distinction between gray matter and white matter is most often applied to central nervous tissue, which has large regions that can be seen with the unaided eye. When looking at peripheral structures, often a microscope is used and the tissue is stained with artificial colors. That is not to say that central nervous tissue cannot be stained and viewed under a microscope, but unstained tissue is most likely from the CNS—for example, a frontal section of the brain or cross section of the spinal cord.
Regardless of the appearance of stained or unstained tissue, the cell bodies of neurons or axons can be located in discrete anatomical structures that need to be named. Those names are specific to whether the structure is central or peripheral. A localized collection of neuron cell bodies in the CNS is referred to as a nucleus. In the PNS, a cluster of neuron cell bodies is referred to as a ganglion.
Terminology applied to bundles of axons also differs depending on location. A bundle of axons, or fibers, found in the CNS is called a tract whereas the same thing in the PNS would be called a nerve. There is an important point to make about these terms, which is that they can both be used to refer to the same bundle of axons. When those axons are in the PNS, the term is nerve, but if they are CNS, the term is tract. The most obvious example of this is the axons that project from the retina into the brain. Those axons are called the optic nerve as they leave the eye, but when they are inside the cranium, they are referred to as the optic tract. There is a specific place where the name changes, which is the optic chiasm, but they are still the same axons (Figure 8.8). Table 8.1 helps to clarify which of these terms apply to the central or peripheral nervous systems.
CNS | PNS | |
Group of Neuron Cell Bodies (i.e., gray matter) | Nucleus | Ganglion |
Bundle of Axons (i.e., white matter) | Tract | Nerve |
The Central Nervous System
The brain and the spinal cord are the central nervous system, and they represent the main organs of the nervous system. The spinal cord is a single structure, whereas the adult brain is described in terms of four major regions: the cerebrum, the diencephalon, the brain stem, and the cerebellum. A person’s conscious experiences are based on neural activity in the brain. The regulation of homeostasis is governed by a specialized region in the brain. The coordination of reflexes depends on the integration of sensory and motor pathways in the spinal cord.
The Cerebrum
The iconic gray mantle of the human brain, which appears to make up most of the mass of the brain, is the cerebrum (Figure 8.9). The wrinkled portion is the cerebral cortex, and the rest of the structure is beneath that outer covering. There is a large separation between the two sides of the cerebrum called the longitudinal fissure. It separates the cerebrum into two distinct halves, a right and left cerebral hemisphere. Deep within the cerebrum, the white matter of the corpus callosum provides the major pathway for communication between the two hemispheres of the cerebral cortex.
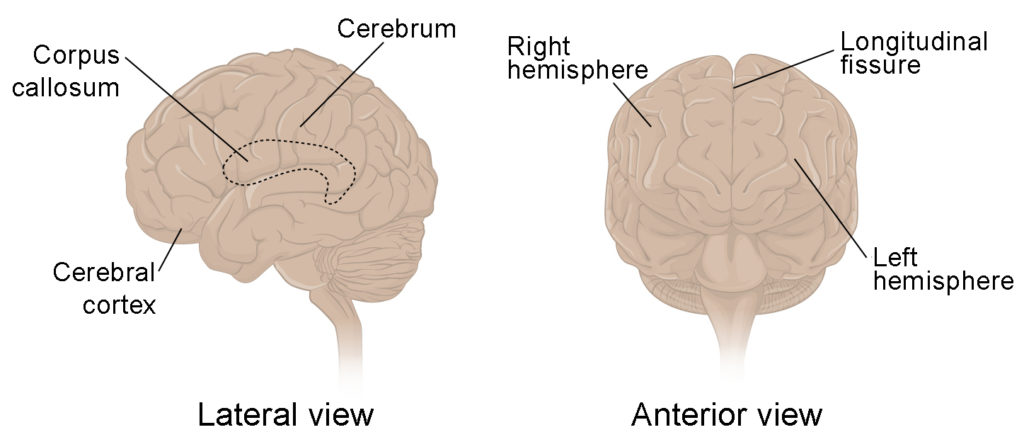
Many of the higher neurological functions, such as memory, emotion, and consciousness, are the result of cerebral function. The complexity of the cerebrum is different across vertebrate species. The cerebrum of the most primitive vertebrates is not much more than the connection for the sense of smell. In mammals, the cerebrum comprises the outer gray matter that is the cortex (from the Latin word meaning “bark of a tree”) and several deep nuclei that belong to three important functional groups. The basal nuclei are responsible for cognitive processing, the most important function being that associated with planning movements. The basal forebrain contains nuclei that are important in learning and memory. The limbic cortex is the region of the cerebral cortex that is part of the limbic system, a collection of structures involved in emotion, memory, and behavior.
Cerebral Cortex
The cerebrum is covered by a continuous layer of gray matter that wraps around either side of the forebrain—the cerebral cortex. This thin, extensive region of wrinkled gray matter is responsible for the higher functions of the nervous system. A gyrus (plural = gyri) is the ridge of one of those wrinkles, and a sulcus (plural = sulci) is the groove between two gyri. The pattern of these folds of tissue indicates specific regions of the cerebral cortex.
The head is limited by the size of the birth canal, and the brain must fit inside the cranial cavity of the skull. Extensive folding in the cerebral cortex enables more gray matter to fit into this limited space. If the gray matter of the cortex were peeled off of the cerebrum and laid out flat, its surface area would be roughly equal to one square meter.
The folding of the cortex maximizes the amount of gray matter in the cranial cavity. The surface of the brain can be mapped on the basis of the locations of large gyri and sulci. Using these landmarks, the cortex can be separated into four major regions, or lobes (Figure 8.10). The lateral sulcus that separates the temporal lobe from the other regions is one such landmark. Superior to the lateral sulcus are the parietal lobe and frontal lobe, which are separated from each other by the central sulcus. The posterior region of the cortex is the occipital lobe, which has no obvious anatomical border between it and the parietal or temporal lobes on the lateral surface of the brain. From the medial surface, an obvious landmark separating the parietal and occipital lobes is called the parieto-occipital sulcus. The fact that there is no obvious anatomical border between these lobes is consistent with the functions of these regions being interrelated.
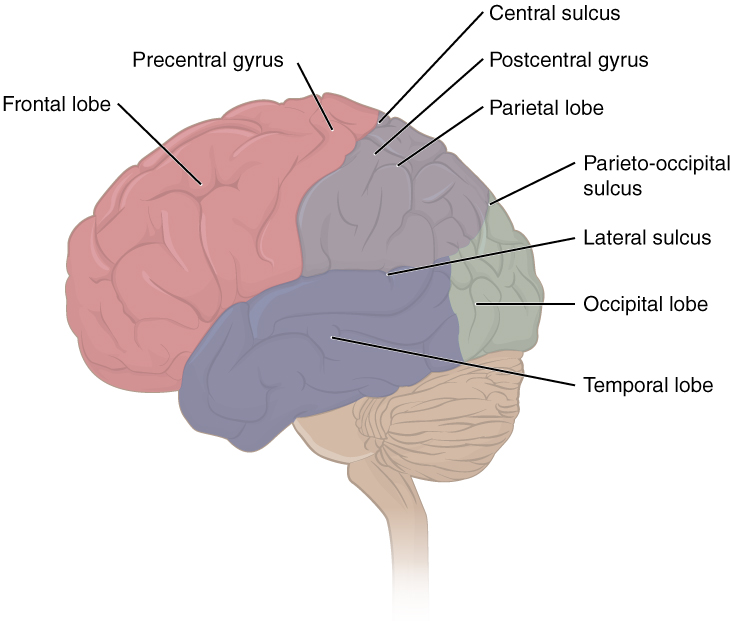
Different regions of the cerebral cortex can be associated with particular functions, a concept known as localization of function. In the early 1900s, a German neuroscientist named Korbinian Brodmann performed an extensive study of the microscopic anatomy—the cytoarchitecture—of the cerebral cortex and divided the cortex into 52 separate regions on the basis of the histology of the cortex. His work resulted in a system of classification known as Brodmann’s areas, which is still used today to describe the anatomical distinctions within the cortex (Figure 8.11). The results from Brodmann’s work on the anatomy align very well with the functional differences within the cortex. Areas 17 and 18 in the occipital lobe are responsible for primary visual perception. That visual information is complex, so it is processed in the temporal and parietal lobes as well.
The temporal lobe is associated with primary auditory sensation, known as Brodmann’s areas 41 and 42 in the superior temporal lobe. Because regions of the temporal lobe are part of the limbic system, memory is an important function associated with that lobe. Memory is essentially a sensory function; memories are recalled sensations such as the smell of Mom’s baking or the sound of a barking dog. Even memories of movement are really the memory of sensory feedback from those movements, such as stretching muscles or the movement of the skin around a joint. Structures in the temporal lobe are responsible for establishing long-term memory, but the ultimate location of those memories is usually in the region in which the sensory perception was processed.
The main sensation associated with the parietal lobe is somatosensation, meaning the general sensations associated with the body. Posterior to the central sulcus is the postcentral gyrus, the primary somatosensory cortex, which is identified as Brodmann’s areas 1, 2, and 3. All of the tactile senses are processed in this area, including touch, pressure, tickle, pain, itch, and vibration, as well as more general senses of the body such as proprioception and kinesthesia, which are the senses of body position and movement, respectively.
Anterior to the central sulcus is the frontal lobe, which is primarily associated with motor functions. The precentral gyrus is the primary motor cortex. Cells from this region of the cerebral cortex are the upper motor neurons that instruct cells in the spinal cord to move skeletal muscles. Anterior to this region are a few areas that are associated with planned movements. The premotor area is responsible for thinking of a movement to be made. The frontal eye fields are important in eliciting eye movements and in attending to visual stimuli. Broca’s area is responsible for the production of language, or controlling movements responsible for speech; in the vast majority of people, it is located only on the left side. Anterior to these regions is the prefrontal lobe, which serves cognitive functions that can be the basis of personality, short-term memory, and consciousness.
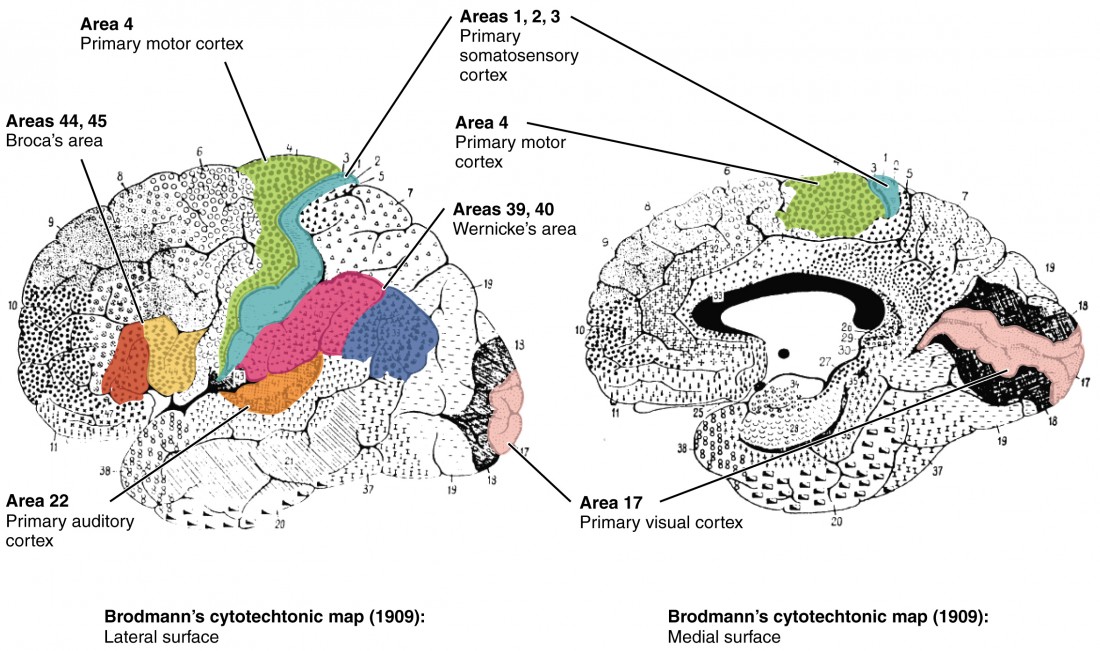
The Diencephalon
The etymology of the word diencephalon translates to “through brain.” It is the connection between the cerebrum and the rest of the nervous system, with one exception, olfaction (the sense of smell), which connects directly with the cerebrum. The rest of the brain, the spinal cord, and the PNS all send information to the cerebrum through the diencephalon. Output from the cerebrum passes through the diencephalon.
The diencephalon is deep beneath the cerebrum and constitutes the walls of the third ventricle. The diencephalon can be described as any region of the brain with “thalamus” in its name. The two major regions of the diencephalon are the thalamus itself and the hypothalamus (Figure 8.12). There are other structures, such as the epithalamus, which contains the pineal gland.
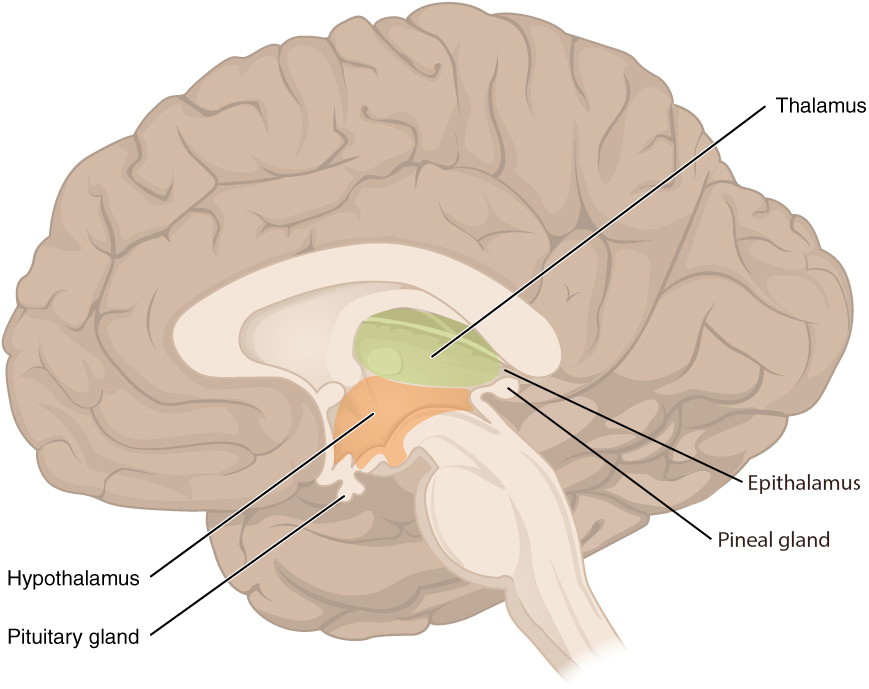
The thalamus is a collection of nuclei that relay information between the cerebral cortex and the periphery, spinal cord, or brain stem. All sensory information, except for the sense of smell, passes through the thalamus before processing by the cortex. Axons from the peripheral sensory organs, or intermediate nuclei, synapse in the thalamus, and thalamic neurons project directly to the cerebrum. The thalamus does not just pass the information on, it also processes that information. For example, the portion of the thalamus that receives visual information will influence what visual stimuli are important, or what receives attention. The cerebrum also sends information down to the thalamus, which usually communicates motor commands. This involves interactions with the cerebellum and other nuclei in the brain stem.
Inferior and slightly anterior to the thalamus is the hypothalamus, the other major region of the diencephalon. The hypothalamus is a collection of nuclei that are largely involved in regulating homeostasis. The hypothalamus is the executive region in charge of the autonomic nervous system and the endocrine system through its regulation of the anterior pituitary gland. Other parts of the hypothalamus are involved in memory and emotion as part of the limbic system.
Brain Stem
The midbrain and hindbrain (composed of the pons and the medulla oblongata) are collectively referred to as the brain stem (Figure 8.13). The structure emerges from the ventral surface of the forebrain as a tapering cone that connects the brain to the spinal cord. Attached to the brain stem, but considered a separate region of the adult brain, is the cerebellum. The midbrain coordinates sensory representations of the visual, auditory, and somatosensory perceptual spaces. The pons is the main connection with the cerebellum. The pons and the medulla regulate several crucial functions, including the cardiovascular and respiratory systems and rates.
The cranial nerves connect through the brain stem and provide the brain with the sensory input and motor output associated with the head and neck, including most of the special senses. The major ascending and descending pathways between the spinal cord and brain, specifically the cerebrum, pass through the brain stem.
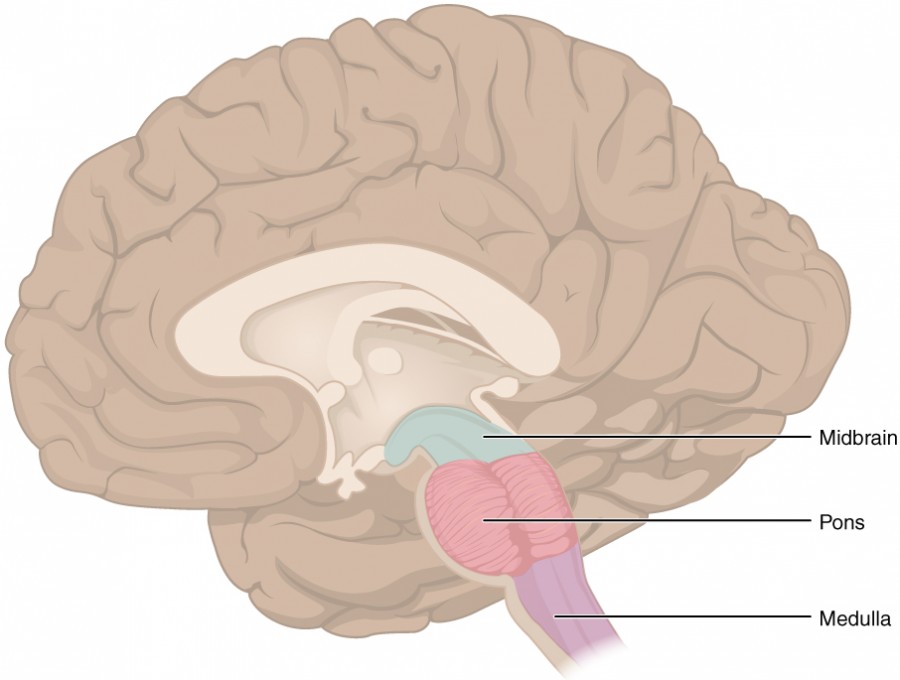
The Cerebellum
The cerebellum, as the name suggests, is the “little brain.” It is covered in gyri and sulci like the cerebrum, and looks like a miniature version of that part of the brain (Figure 8.14). The cerebellum is largely responsible for comparing information from the cerebrum with sensory feedback from the periphery through the spinal cord. It accounts for approximately 10 percent of the mass of the brain.
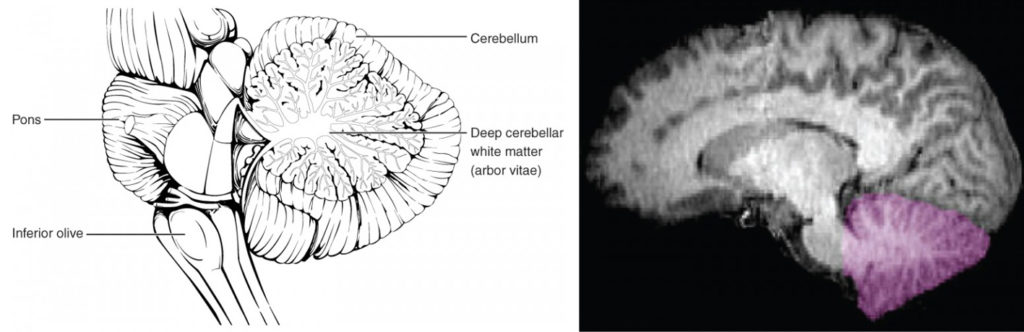
When the cerebrum sends motor commands sent to the spinal cord, a copy is sent to the cerebellum, as well. A copy of sensory information from the periphery, which enters through spinal or cranial nerves, is also sent to the cerebellum. If the primary motor cortex of the frontal lobe sends a command down to the spinal cord to initiate walking, a copy of that instruction is sent to the cerebellum. Sensory feedback from the muscles and joints, proprioceptive information about the movements of walking, and sensations of balance are sent to the cerebellum and the cerebellum compares them. If walking is not coordinated, perhaps because the ground is uneven or a strong wind is blowing, then the cerebellum sends out a corrective command to compensate for the difference between the original cortical command and the sensory feedback. The output of the cerebellum is into the midbrain, which then sends a descending input to the spinal cord to correct the messages going to skeletal muscles.
The Spinal Cord
The description of the CNS is concentrated on the structures of the brain, but the spinal cord is another major organ of the system. The anterior midline is marked by the anterior median fissure, and the posterior midline is marked by the posterior median sulcus. Axons enter the posterior side through the dorsal (posterior) nerve root. The axons emerging from the anterior side do so through the ventral (anterior) nerve root. On the whole, the posterior regions are responsible for sensory functions and the anterior regions are associated with motor functions. Note that it is common to see the terms dorsal (dorsal = “back”) and ventral (ventral = “belly”) used interchangeably with posterior and anterior, particularly in reference to nerves and the structures of the spinal cord. You should learn to be comfortable with both.
The length of the spinal cord is divided into regions that correspond to the regions of the vertebral column. The name of a spinal cord region corresponds to the level at which spinal nerves pass through the intervertebral foramina. Immediately adjacent to the brain stem is the cervical region, followed by the thoracic, then the lumbar, and finally the sacral region. The spinal cord is not the full length of the vertebral column because the spinal cord does not grow significantly longer after the first or second year, but the skeleton continues to grow. The nerves that emerge from the spinal cord pass through the intervertebral foramina at the respective levels. As the vertebral column grows, these nerves grow with it and result in a long bundle of nerves that resembles a horse’s tail and is named the cauda equina. The sacral spinal cord is at the level of the upper lumbar vertebral bones. The spinal nerves extend from their various levels to the proper level of the vertebral column.
Gray Horns
In cross-section, the gray matter of the spinal cord has the appearance of an ink-blot test, with the spread of the gray matter on one side replicated on the other—a shape reminiscent of a bulbous capital “H.” As shown in Figure 8.15, the gray matter is subdivided into regions that are referred to as horns. The posterior horn is responsible for sensory processing. The anterior horn sends out motor signals to the skeletal muscles. The lateral horn, which is only found in the thoracic, upper lumbar, and sacral regions, is the central component of the sympathetic division of the autonomic nervous system.
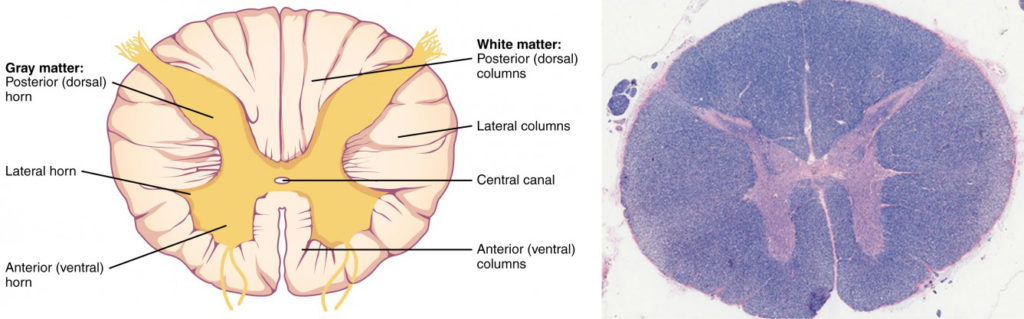
White Columns
Just as the gray matter is separated into horns, the white matter of the spinal cord is separated into columns. Ascending tracts of nervous system fibers in these columns carry sensory information up to the brain, whereas descending tracts carry motor commands from the brain. Looking at the spinal cord longitudinally, the columns extend along its length as continuous bands of white matter. Between the two posterior horns of gray matter are the posterior columns. Between the two anterior horns, and bounded by the axons of motor neurons emerging from that gray matter area, are the anterior columns. The white matter on either side of the spinal cord, between the posterior horn and the axons of the anterior horn neurons, are the lateral columns. The posterior columns are composed of axons of ascending tracts. The anterior and lateral columns are composed of many different groups of axons of both ascending and descending tracts—the latter carrying motor commands down from the brain to the spinal cord to control output to the periphery.
Protective Coverings of the Brain and Spinal Cord
The outer surface of the CNS is covered by a series of membranes composed of connective tissue called the meninges, which protect the brain. The dura mater is a thick fibrous layer and a strong protective sheath over the entire brain and spinal cord. It is anchored to the inner surface of the cranium and vertebral cavity. The arachnoid mater is a membrane of thin fibrous tissue that forms a loose sac around the CNS. Beneath the arachnoid is a thin, filamentous mesh called the arachnoid trabeculae, which looks like a spider web, giving this layer its name. Directly adjacent to the surface of the CNS is the pia mater, a thin fibrous membrane that follows the convolutions of gyri and sulci in the cerebral cortex and fits into other grooves and indentations (Figure 8.16).
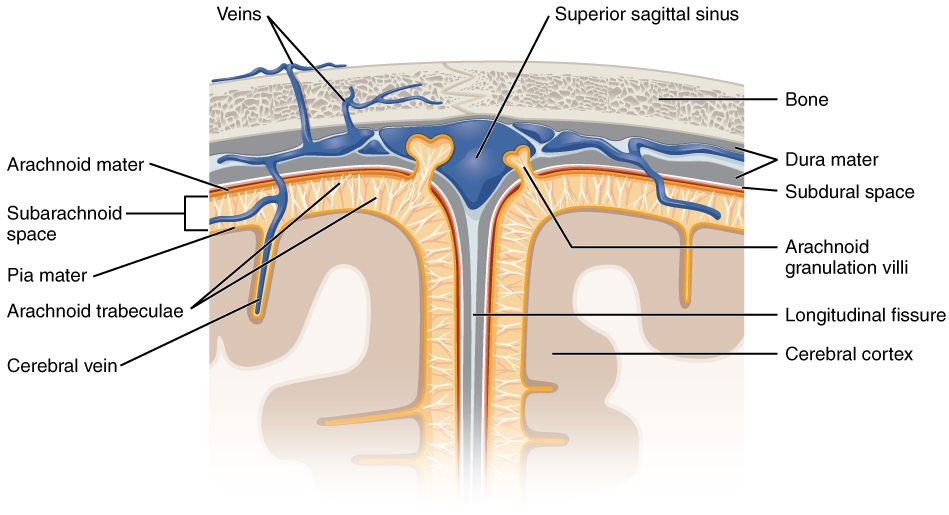
Dura Mater
Like a thick cap covering the brain, the dura mater is a tough outer covering. The name comes from the Latin for “tough mother” to represent its physically protective role. It encloses the entire CNS and the major blood vessels that enter the cranium and vertebral cavity. It is directly attached to the inner surface of the bones of the cranium and to the very end of the vertebral cavity. There are infoldings of the dura that fit into large crevasses of the brain. Two infoldings go through the midline separations of the cerebrum and cerebellum; one forms a shelf-like tent between the occipital lobes of the cerebrum and the cerebellum, and the other surrounds the pituitary gland. The dura also surrounds and supports the venous sinuses.
After passing through the CNS, blood returns to the circulation through a series of dural sinuses and veins (Figure 8.17). The superior sagittal sinus runs in the groove of the longitudinal fissure, where it absorbs CSF from the meninges. The superior sagittal sinus drains to the confluence of sinuses, along with the occipital sinuses and straight sinus, to then drain into the transverse sinuses. The transverse sinuses connect to the sigmoid sinuses, which then connect to the jugular veins. From there, the blood continues toward the heart to be pumped to the lungs for reoxygenation.
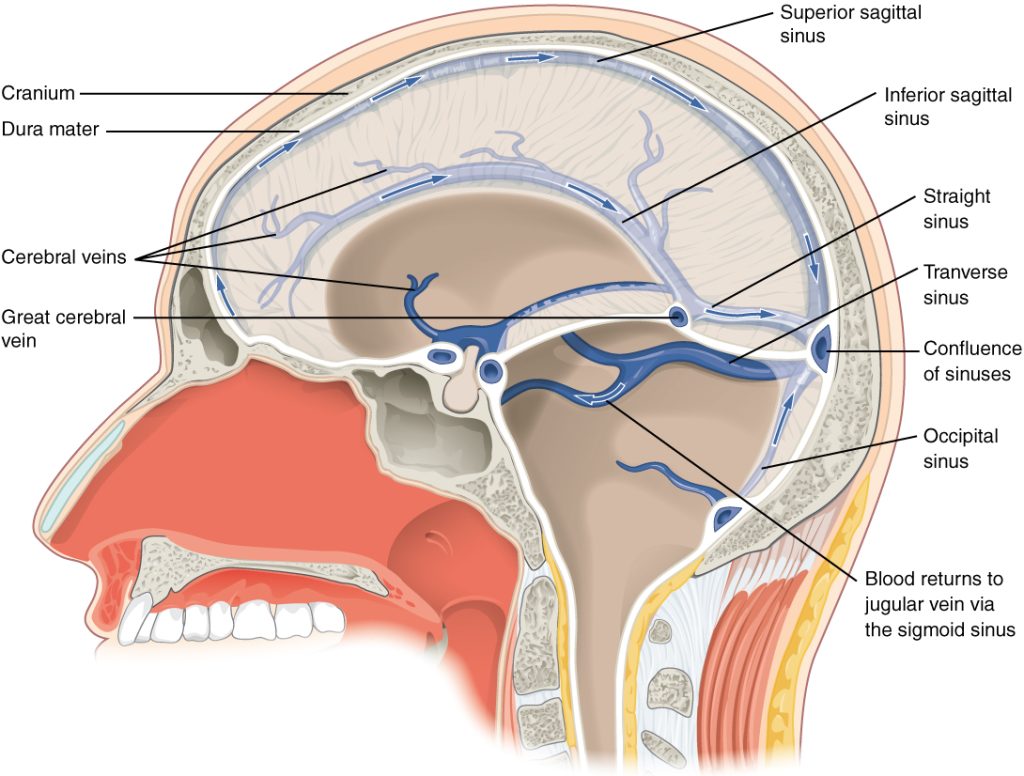
Arachnoid Mater
The middle layer of the meninges is the arachnoid, named for the spider-web–like trabeculae between it and the pia mater. The arachnoid defines a sac-like enclosure around the CNS. The trabeculae are found in the subarachnoid space, which is filled with circulating CSF. The arachnoid emerges into the dural sinuses as the arachnoid granulations, where the CSF is filtered back into the blood for drainage from the nervous system (Figure 8.16).
The subarachnoid space is filled with circulating CSF, which also provides a liquid cushion to the brain and spinal cord. Similar to clinical blood work, a sample of CSF can be withdrawn to find chemical evidence of neuropathology or metabolic traces of the biochemical functions of nervous tissue.
Pia Mater
The outer surface of the CNS is covered in the thin fibrous membrane of the pia mater. It is thought to have a continuous layer of cells providing a fluid-impermeable membrane. The name pia mater comes from the Latin for “tender mother,” suggesting the thin membrane is a gentle covering for the brain. The pia extends into every convolution of the CNS, lining the inside of the sulci in the cerebral and cerebellar cortices. Blood vessels that are nourishing the central nervous tissue are between the pia mater and the nervous tissue.
The Ventricular System
Cerebrospinal fluid (CSF) circulates throughout and around the CNS. In other tissues, water and small molecules are filtered through capillaries as the major contributor to the interstitial fluid. In the brain, CSF is produced in special structures to perfuse through the nervous tissue of the CNS and is continuous with the interstitial fluid. Specifically, CSF circulates to remove metabolic wastes from the interstitial fluids of nervous tissues and return them to the blood stream. The ventricles are the open spaces within the brain where CSF circulates. In some of these spaces, CSF is produced by filtering of the blood that is performed by a specialized membrane known as a choroid plexus. The CSF circulates through all of the ventricles to eventually emerge into the subarachnoid space where it will be reabsorbed into the blood.
There are four ventricles within the brain. The first two are named the lateral ventricles and are deep within the cerebrum. These ventricles are connected to the third ventricle by two openings called the interventricular foramina. The third ventricle is the space between the left and right sides of the diencephalon, which opens into the cerebral aqueduct that passes through the midbrain. The aqueduct opens into the fourth ventricle, which is the space between the cerebellum and the pons and upper medulla (Figure 8.18).
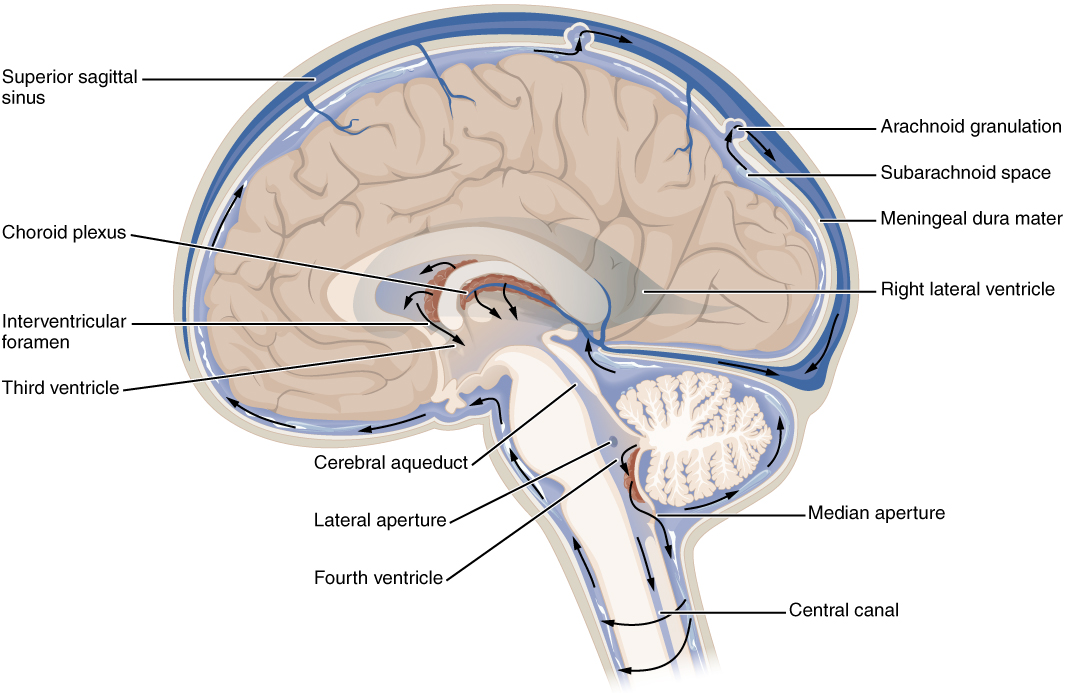
The ventricular system opens up to the subarachnoid space from the fourth ventricle. The single median aperture and the pair of lateral apertures connect to the subarachnoid space so that CSF can flow through the ventricles and around the outside of the CNS. Cerebrospinal fluid is produced within the ventricles by a type of specialized membrane called a choroid plexus. Ependymal cells (one of the types of glial cells described in the introduction to the nervous system) surround blood capillaries and filter the blood to make CSF. The fluid is a clear solution with a limited amount of the constituents of blood. It is essentially water, small molecules, and electrolytes. Oxygen and carbon dioxide are dissolved into the CSF, as they are in blood, and can diffuse between the fluid and the nervous tissue.
A very small amount of CSF is filtered at any one of the plexuses, for a total of about 500 milliliters daily, but it is continuously made and pulses through the ventricular system, keeping the fluid moving. Within the subarachnoid space, the CSF flows around all of the CNS, providing two important functions. As with elsewhere in its circulation, the CSF picks up metabolic wastes from the nervous tissue and moves it out of the CNS. It also acts as a liquid cushion for the brain and spinal cord. By surrounding the entire system in the subarachnoid space, it provides a thin buffer around the organs within the strong, protective dura mater. The arachnoid granulations are outpocketings of the arachnoid membrane into the dural sinuses so that CSF can be reabsorbed into the blood, along with the metabolic wastes. From the dural sinuses, blood drains out of the head and neck through the jugular veins, along with the rest of the circulation for blood, to be reoxygenated by the lungs and wastes to be filtered out by the kidneys.
The Peripheral Nervous System
The PNS is not as contained as the CNS because it is defined as everything that is not the CNS. Some peripheral structures are incorporated into the other organs of the body. In describing the anatomy of the PNS, it is necessary to describe the common structures, the nerves and the ganglia, as they are found in various parts of the body. Many of the neural structures that are incorporated into other organs are features of the digestive system; these structures are known as the enteric nervous system and are a special subset of the PNS.
Ganglia
A ganglion is a group of neuron cell bodies in the periphery. Ganglia can be categorized, for the most part, as either sensory ganglia or autonomic ganglia, referring to their primary functions. The most common type of sensory ganglion is a dorsal (posterior) root ganglion. These ganglia are the cell bodies of neurons with axons that are sensory endings in the periphery, such as in the skin, and that extend into the CNS through the dorsal nerve root of the spinal cord (Figure 8.19). The ganglion is an enlargement of the nerve root. Under microscopic inspection, it can be seen to include the cell bodies of the neurons, as well as bundles of fibers that are the posterior nerve root (Figure 8.20).

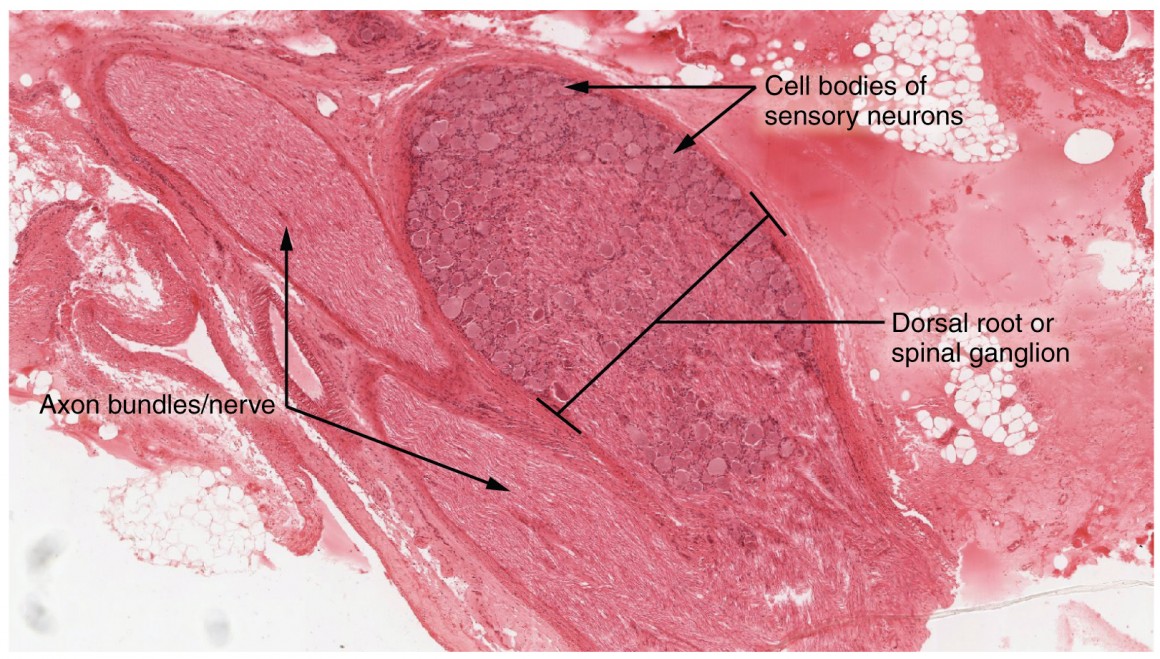
Another type of sensory ganglion is a cranial nerve ganglion. This is analogous to the dorsal root ganglion, except that it is associated with a cranial nerve instead of a spinal nerve, which is what the dorsal root ganglion is associated with. The roots of cranial nerves are within the cranium, whereas the ganglia are outside the skull.
The other major category of ganglia are those of the autonomic nervous system, which is divided into the sympathetic and parasympathetic nervous systems. The sympathetic chain ganglia constitute a row of ganglia along the vertebral column that receive central input from the lateral horn of the thoracic and upper lumbar spinal cord. There are also surrounding paravertebral ganglia and prevertebral ganglia, which are located outside of the chain but have similar functions. The neurons of the chain, paravertebral, and prevertebral ganglia then project to organs in the head and neck, thoracic, abdominal, and pelvic cavities to regulate the sympathetic aspect of homeostatic mechanisms.
Another group of autonomic ganglia are the terminal ganglia that receive input from cranial nerves or sacral spinal nerves and are responsible for regulating the parasympathetic aspect of homeostatic mechanisms. These two sets of ganglia, sympathetic and parasympathetic, often project to the same organs—one input from the chain ganglia and one input from a terminal ganglion—to regulate the overall function of an organ. For example, the heart receives two inputs such as these; one increases heart rate, and the other decreases it.
Nerves
Bundles of axons in the PNS are referred to as nerves. These structures in the periphery are different than the central counterpart, called a tract. Nerves are composed of more than just nervous tissue. They have connective tissues invested in their structure, as well as blood vessels supplying the tissues with nourishment. The outer surface of a nerve is a surrounding layer of fibrous connective tissue called the epineurium. Within the nerve, axons are further bundled into fascicles, which are each surrounded by their own layer of fibrous connective tissue called perineurium. Finally, individual axons are surrounded by loose connective tissue called the endoneurium (Figure 8.21). These three layers are similar to the connective tissue sheaths for muscles. Nerves are associated with the region of the CNS to which they are connected, either as cranial nerves connected to the brain or spinal nerves connected to the spinal cord.
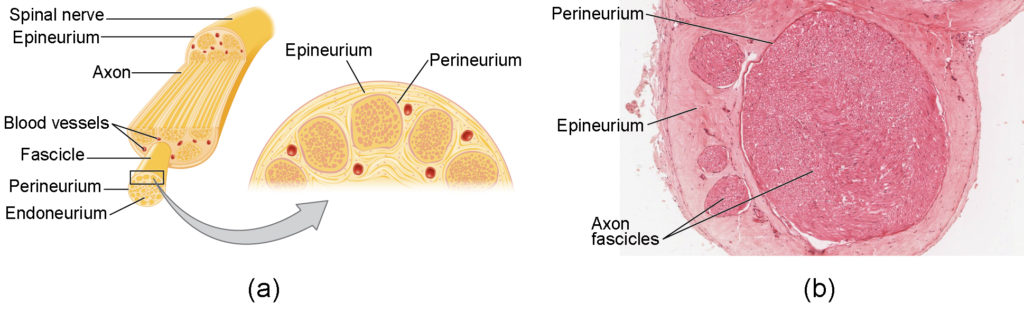
Cranial Nerves
The nerves attached to the brain are the cranial nerves, which are primarily responsible for the sensory and motor functions of the head and neck (one of these nerves targets organs in the thoracic and abdominal cavities as part of the parasympathetic nervous system). There are twelve cranial nerves, which are designated CN I through CN XII for “Cranial Nerve,” using Roman numerals for 1 through 12 (Figure 8.22). They can be classified as sensory nerves, motor nerves, or a combination of both, meaning that the axons in these nerves originate out of sensory ganglia external to the cranium or motor nuclei within the brain stem. Sensory axons enter the brain to synapse in a nucleus. Motor axons connect to skeletal muscles of the head or neck. Three of the nerves are solely composed of sensory fibers; five are strictly motor; and the remaining four are mixed nerves.
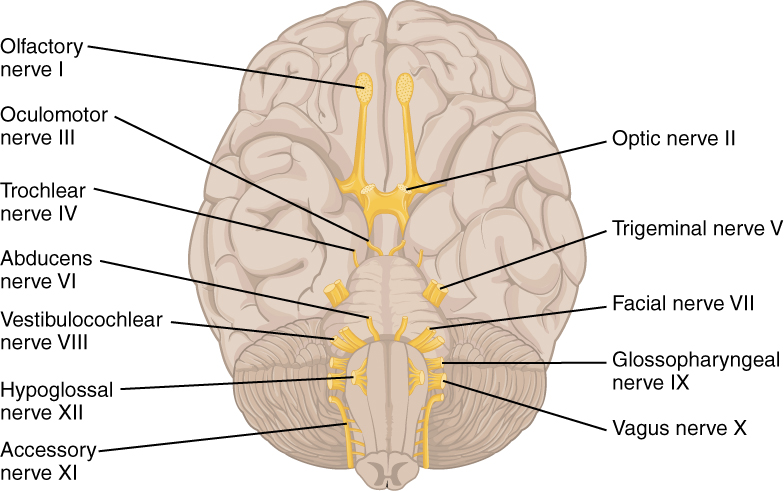
Learning the cranial nerves is a tradition in anatomy courses, and students have always used mnemonic devices to remember the nerve names. A traditional mnemonic is the rhyming couplet, “On Old Olympus’ Towering Tops/A Finn And German Viewed Some Hops,” in which the initial letter of each word corresponds to the initial letter in the name of each nerve. The names of the nerves have changed over the years to reflect current usage and more accurate naming. An exercise to help learn this sort of information is to generate a mnemonic using words that have personal significance. The names of the cranial nerves are listed in Table 8.2 along with a brief description of their function, their source (sensory ganglion or motor nucleus), and their target (sensory nucleus or skeletal muscle).
The olfactory nerve and optic nerve are responsible for the sense of smell and vision, respectively. The oculomotor nerve is responsible for eye movements by controlling four of the extraocular muscles. It is also responsible for lifting the upper eyelid when the eyes point up, and for pupillary constriction. The trochlear nerve and the abducens nerve are both responsible for eye movement, but do so by controlling different extraocular muscles. The trigeminal nerve is responsible for cutaneous sensations of the face and controlling the muscles of mastication. The facial nerve is responsible for the muscles involved in facial expressions, as well as part of the sense of taste and the production of saliva. The vestibulocochlear nerve is responsible for the senses of hearing and balance. The glossopharyngeal nerve is responsible for controlling muscles in the oral cavity and upper throat, as well as part of the sense of taste and the production of saliva. The vagus nerve is responsible for contributing to homeostatic control of the organs of the thoracic and upper abdominal cavities. The accessory nerve is responsible for controlling the muscles of the neck, along with cervical spinal nerves. The hypoglossal nerve is responsible for controlling the muscles of the lower throat and tongue.
Four of the cranial nerves also contain autonomic fibers. The oculomotor, facial, and glossopharyngeal nerves contain fibers that contact autonomic ganglia. The oculomotor fibers initiate pupillary constriction, whereas the facial and glossopharyngeal fibers both initiate salivation. The vagus nerve primarily targets autonomic ganglia in the thoracic and upper abdominal cavities.
Another important aspect of the cranial nerves that lends itself to a mnemonic is the functional role each nerve plays. The nerves fall into one of three basic groups. They are sensory, motor, or both (see Table 8.2). The sentence, “Some Say Marry Money But My Brother Says Brains Beauty Matter More,” corresponds to the basic function of each nerve. The first, second, and eighth nerves are purely sensory: the olfactory (CNI), optic (CNII), and vestibulocochlear (CNVIII) nerves. The three eye-movement nerves are all motor: the oculomotor (CNIII), trochlear (CNIV), and abducens (CNVI). The accessory (CNXI) and hypoglossal (CNXII) nerves are also strictly motor. The remainder of the nerves contain both sensory and motor fibers. They are the trigeminal (CNV), facial (CNVII), glossopharyngeal (CNIX), and vagus (CNX) nerves. The nerves that convey both are often related to each other. The trigeminal and facial nerves both concern the face; one concerns the sensations and the other concerns the muscle movements. The facial and glossopharyngeal nerves are both responsible for conveying gustatory, or taste, sensations as well as controlling salivary glands. The vagus nerve is involved in visceral responses to taste, namely the gag reflex. This is not an exhaustive list of what these combination nerves do, but there is a thread of relation between them.
Mnemonic | # | Name | Function (S/M/B) | Central connection (nuclei) | Peripheral connection (ganglion or muscle) |
On | I | Olfactory | Smell (S) | Olfactory bulb | Olfactory epithelium |
Old | II | Optic | Vision (S) | Hypothalamus/thalamus/midbrain | Retina (retinal ganglion cells) |
Olympus | III | Oculomotor | Eye movements (M) | Oculomotor nucleus | Extraocular muscles (other 4), levator palpebrae superioris, ciliary ganglion (autonomic) |
Towering | IV | Trochlear | Eye movements (M) | Trochlear nucleus | Superior oblique muscle |
Tops | V | Trigeminal | Sensory/motor—face (B) | Trigeminal nuclei in the midbrain, pons, and medulla | Trigemal |
A | VI | Abducens | Eye movements (M) | Abducens nucleus | Lateral rectus muscle |
Finn | VII | Facial | Motor—face, Taste (B) | Facial nucleus, solitary nucleus, superior salivatory nucleus | Facial muscles, Geniculate ganglion, Pterygopalatine ganglion (autonomic) |
And | VIII | Vestibulocochlear | Hearing/balance (S) | Cochlearn nucleus, Vestibular nucleus/cerebellum | Spiral ganglion (hearing), Vestibular ganglion (balance) |
German | IX | Glossopharyngeal | Motor—throat Taste (B) | Solitary nucleus, inferior salivatory nucleus, nucleus ambiguus | Pharyngeal muscles, Geniculate ganglion, Otic ganglion (autonomic) |
Viewed | X | Vagus | Motor/sensory—viscera (autonomic) | Medulla | Terminal ganglia serving thoracic and upper abdominal organs (heart and small intestines) |
Some | XI | Accessory | Motor—head and neck (M) | Spinal accessory nucleus | Neck muscles |
Hops | XII | Hypoglossal | Motor—lower throat (M) | Hypoglossal nucleus | Muscles of the larynx and lower pharynx |
Spinal Nerves
The nerves connected to the spinal cord are the spinal nerves. The arrangement of these nerves is much more regular than that of the cranial nerves. All of the spinal nerves are combined sensory and motor axons that separate into two nerve roots. The sensory axons enter the spinal cord as the dorsal nerve root. The motor fibers, both somatic and autonomic, emerge as the ventral nerve root. The dorsal root ganglion for each nerve is an enlargement of the spinal nerve (see Figure 8.19).
There are 31 spinal nerves, named for the level of the spinal cord at which each one emerges. There are eight pairs of cervical nerves designated C1 to C8, twelve thoracic nerves designated T1 to T12, five pairs of lumbar nerves designated L1 to L5, five pairs of sacral nerves designated S1 to S5, and one pair of coccygeal nerves. The nerves are numbered from the superior to inferior positions, and each emerges from the vertebral column through the intervertebral foramen at its level.
Spinal nerves extend outward from the vertebral column to enervate the periphery. The nerves in the periphery are not straight continuations of the spinal nerves, but rather the reorganization of the axons in those nerves to follow different courses. Axons from different spinal nerves will come together into a systemic nerve. This occurs at four places along the length of the vertebral column, each identified as a nerve plexus, whereas the other spinal nerves directly correspond to nerves at their respective levels. In this instance, the word plexus is used to describe networks of nerve fibers with no associated cell bodies.
Of the four nerve plexuses, two are found at the cervical level, one at the lumbar level, and one at the sacral level (Figure 8.23). The cervical plexus is composed of axons from spinal nerves C1 through C5 and branches into nerves in the posterior neck and head, as well as the phrenic nerve, which connects to the diaphragm at the base of the thoracic cavity. The other plexus from the cervical level is the brachial plexus. Spinal nerves C4 through T1 reorganize through this plexus to give rise to the nerves of the arms, as the name brachial suggests. A large nerve from this plexus is the radial nerve from which the axillary nerve branches to go to the armpit region. The radial nerve continues through the arm and is paralleled by the ulnar nerve and the median nerve. The lumbar plexus arises from all the lumbar spinal nerves and gives rise to nerves enervating the pelvic region and the anterior leg. The femoral nerve is one of the major nerves from this plexus.The sacral plexus comes from the lower lumbar nerves L4 and L5 and the sacral nerves S1 to S4. The most significant systemic nerve to come from this plexus is the sciatic nerve, which is a combination of the tibial nerve and the fibular nerve.
These plexuses are described as arising from spinal nerves and giving rise to certain systemic nerves, but they contain fibers that serve sensory functions or fibers that serve motor functions. This means that some fibers extend from cutaneous or other peripheral sensory surfaces and send action potentials into the CNS. Those are axons of sensory neurons in the dorsal root ganglia that enter the spinal cord through the dorsal nerve root. Other fibers are the axons of motor neurons of the anterior horn of the spinal cord, which emerge in the ventral nerve root and send action potentials to cause skeletal muscles to contract in their target regions. For example, the radial nerve contains fibers of cutaneous sensation in the arm, as well as motor fibers that move muscles in the arm.
Spinal nerves of the thoracic region, T2 through T11, are not part of the plexuses but rather emerge and give rise to the intercostal nerves found between the ribs, which articulate with the vertebrae surrounding the spinal nerve.
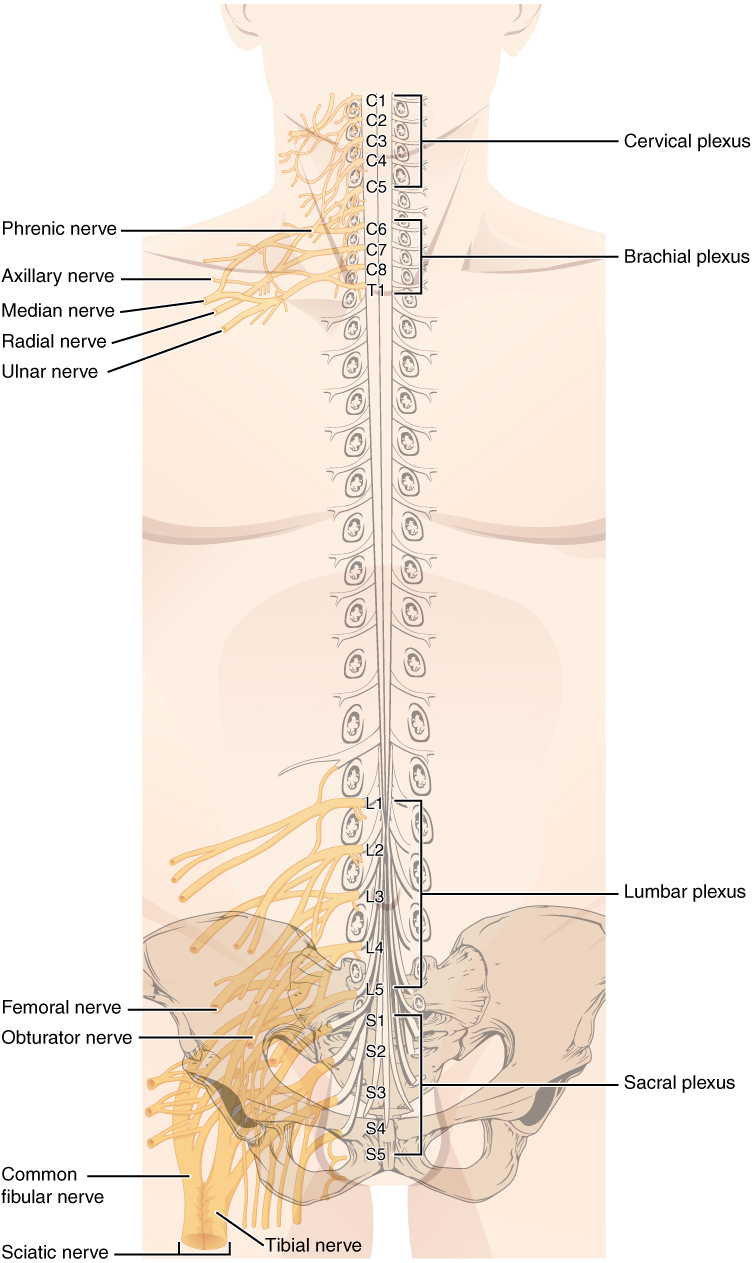
Unless otherwise indicated, this chapter contains material adapted from chapters 13.1-13.4 in Anatomy and Physiology 2e (on OpenStax), by Betts, et al. and is used under a a CC BY 4.0 international license. Download and access OpenStax Anatomy and Physiology for free at https://openstax.org/details/books/anatomy-and-physiology-2e/