Inbreeding
Walter R. Fehr and Walter P. Suza
Readings:
- Chapter 8: Inbreeding [pdf], Principles of Cultivar Development. Vol. 1: Theory and Technique, by Walter R. Fehr (Access the full book)
- Chapters 22-27 [pdf], Principles of Cultivar Development. Vol. 1: Theory and Technique, by Walter R. Fehr
Introduction
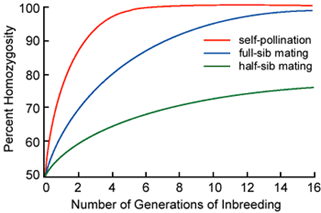
Inbreeding for the development of homozygous and homogeneous lines is only important for the development of pure-line and hybrid cultivars. It is not used for the development of clonal or synthetic cultivars because self-pollination results in severe inbreeding depression.
It is possible to carry out inbreeding by half-sib or full-sib mating, instead of self-pollination. However, the number of generations required to reach homozygosity is more than through the use of self-pollination. As a result, half-sib or full-sib matings rarely are used to develop inbred lines for cultivar development. The chart in figure 1 illustrates the number of generations it may take to attain complete homozygosity by different mating systems.
Factors influencing the choice of methods for obtaining pure lines/inbred lines
Environments available
One of the key factors that influences the method of advancing a population from the F1 to later generations is the environments available to the breeder. The environment for which a breeder is developing new cultivars will be referred to as the local environment. In most breeding programs today, a breeder will use off-season nurseries for generation advance, which include greenhouses, growth chambers, and locations where the environment is not the same as the local environment. For example, the wheat cultivar Select was developed by breeders in South Dakota for their local environment [JPR 5:196-201 (2011)]. For generation advancement of the population from which the cultivar was developed, the breeders used Yuma, Arizona, for the off-season nursery. The possibility of effectively selecting among plants or lines in Yuma for traits of interest would influence how a population would be planted and harvested in Yuma.
The effectiveness of selection in an off-season nursery is highly dependent on the trait. Although seed yield commonly cannot be evaluated in off-season nurseries, selection for other traits may be possible. For example, the cranberry bean cultivar Bellagio was developed for Michigan [JPR 4:171-174 (2010)]. In the off-season nursery at Isabela, Puerto Rico, the breeders were able to select for upright vine, lodging resistance, good pod load, cranberry bean seed size and color, and freedom from diseases.
The two methods that are most influenced by the environment are the bulk and pedigree. In the bulk method, the seed planted in one generation is obtained by sampling from a bulk of seed harvested the previous generation. The likelihood that a plant will have one of it progeny represented in the sample used for planting is directly associated with the number of seeds the plant produced. An F2 plant that produced 40 seeds would be less likely to be represented in the next generation than an F2 plant that produced 200 seeds. If the number of seeds produced by plants in off-season nurseries was not representative of their production in the local environment, they could be eliminated from the population by use of the bulk method, even though they may have the potential to be a superior new cultivar or inbred. Use of the pedigree method is also highly dependent on the environment of the off-season nursery. If a trait is not adequately expressed in the off-season nursery, selection would be ineffective.
It is common for breeders to use a combination of methods during generation advance. For example, a breeder may select among F2 plants in the local environment, harvest individual F3 plants in an off-season nursery without selection, and resume selection among F3:4 lines the following season in the local environment.
Cost
Half of the genetic variation among individuals in a population will be expressed in the F2 generation. To maximize genetic variation for seed yield and other traits for which selection is not feasible during generation advance, it is ideal to have as many different F2 plants and their progenies represented in the population when lines are selected for evaluation. The methods that can assure that every line derived from a population traces to a different F2 individual are the pedigree, the single-seed procedure of single-seed descent, and the single-hill procedure of single-seed descent. Doubled haploid lines normally trace to individual gametes from an F1 plant, each of which are genetically different. These methods require more labor than the bulk or the multiple-seed procedure of single seed descent. The breeder must decide how to balance cost versus genetic variability in the lines derived from a population.
Generation in which lines are derived
Regardless of the method of generation advance used for a population, the breeder must decide at which generation lines will be derived for extensive testing as a potential cultivar or as an inbred in a hybrid. In selecting parents for mating, genetic differences in their parentage or in their molecular makeup are emphasized; therefore, there would be many heterozygous loci in the F1 hybrid. As a result, complete homozygosity is rarely achieved in plants from which pure-line cultivars or inbreds are derived, with the exception of the doubled haploid method. Instead, the breeder derives lines at a generation when there is an adequate frequency of plants whose progeny will be sufficiently uniform in appearance and homogeneous for major genes of interest.
With multiple segregating loci, the proportion of homozygotes in various selfing generations can be estimated using the following formula: [(2m – 1)2m]n: where m is the number of selfing generations (F2 = 1; F3 = 2) and n is the number of segregating loci. For example, an F1 plant with four independent segregating loci will result in the following frequency of homozygous plants in F2, [(21 – 1)/21]4 1/16 = 6.25%. The expected proportion of completely homozygous plants in F2 and later generations of selfing for different numbers of segregating loci are indicated in table 1. The proportion of homozygotes decreases sharply with increasing heterozygosity in the F1.
Number of segregating loci in the F1 | F2 | F4 | F6 | F8 |
1 | 50.00 | 87.5 | 96.87 | 99.22 |
2 | 25.00 | 76.56 | 93.85 | 98.44 |
3 | 12.50 | 66.99 | 90.91 | 97.67 |
4 | 6.25 | 58.62 | 88.07 | 96.91 |
5 | 3.13 | 51.29 | 85.32 | 96.15 |
10 | 0.01 | 26.31 | 72.79 | 92.45 |
100 | 7.89 x 10-31 | 6.1 x 10-4 | 4.18 | 45.64 |
1000 | 9.33 x 10-302 | 1.02 x 10-58 | 1.63 x 10-14 | 0.04 |
The generation in which a cultivar or inbred line is derived for commercial use is influenced by the degree of homozygosity and homogeneity required and the cost and time involved in each generation of selfing. The number of heterozygous loci is an F1 hybrid generally is very large, which means that only a very small percentage of plants are completely homozygous when lines are derived (Table 1). As a result, pure-line cultivars and inbreds of hybrids rarely are completely homozygous and homogeneous, unless they were derived from doubled haploids. The breeder attempts to achieve the level of homogeneity that is required for commercial acceptance of the cultivar or inbred line. In general, breeders of pure-line cultivars commonly derive cultivars in an earlier generation than breeders responsible for developing inbreds of hybrids. For example, the hard red winter wheat cultivar Camelot originated from an F3-derived line [JPR 3:256-263 (2009)]. In contrast, the maize inbred line GT603 was developed through seven generations of self-pollination [JPR 5:211-214 (2011)]. One reason for seeking more homozygosity and homogeneity in an inbred is to be able to recognize and remove off-type plants in a hybrid seed production field before crossing occurs. A second reason is that if two inbreds are crossed that are not adequately homozygous and homogeneous, undesirable segregation may occur in a commercial hybrid field.
Examples of how the inbreeding methods described in the text have been applied in development of cultivars and germplasm can be found in the Journal of Plant Registrations. In the following examples, the underlined sentences are comments that did not appear in the text.
Example 1: Bulk method combined with mass selection
Information found in JPR 5:151-155 (2011) describes the development of ‘Gadsby’ barley by use of the bulk method and mass selection. The breeders developed Gadsby as a general-purpose barley with resistance to scald.
- Step 1. In 1997, the F2 plants from a cross between the parents H92066001 and TR248 were grown in the field at Lacombe, Alberta, Canada. The plants were inoculated with scald by spreading straw that had been collected from a field infested with the disease the previous year. The F3 seed of the population was harvested in bulk.
- Step 2. For mass selection, the F3 seed was screened over a gravity table and the heavier seed was saved for planting. It was assumed by the breeders that the heavier seed was from F2 plants with resistance to scald and the lighter seed was from susceptible genotypes.
- Step 3. In 1998, the selected F3 seed was planted in the field and the first two steps were repeated again. The same two steps were repeated with the F4 bulk in 1999 and the F5 bulk in 2000.
- Step 4. In 2001, the F6 bulk was grown in 2001 at two locations and a head from each of multiple individual plants was harvested and threshed separately. The progeny of each plant was evaluated in subsequent generations as F6-derived lines; therefore, Gadsby was an F6-derived cultivar.
The purpose of mass selection is to increase the frequency of individuals with a desired trait in a population. By infecting the plants each generation and selecting the heavier ones for planting, the breeders of Gadsby assumed that the frequency of F6 plants with resistance to scald would be greater than if the bulk method had been used without infection or mass selection.
Mass selection also can be used with single-seed descent. For example, if a disease caused resistant plants to look different than susceptible ones, one or a few seeds could be harvested from the resistant ones to plant the next generation.
Mass selection is not expected to be perfect in selecting desirable seeds or plants. In the breeding of Gadsby, some of the susceptible plants may have escaped infection and produced seed as heavy as the resistant ones. As a result, some of the seed planted the next generation may have been from susceptible plants. Even though it is not perfect, it can potentially increase the frequency of desirable individuals in a population.
It is important to recognize that the term “mass selection” when used in connection with inbreeding a population has a very different meaning than when used in connection with recurrent selection (Lessons 13 and 14 of this course). When a population is self-pollinated, as in the case of Gadsby barley, the frequency of homozygous individuals increases each generation of mass selection. When mass selection is carried out for recurrent selection, selected plants are intercrossed, which maintains the heterozygosity of individuals.
Example 2: Single-seed procedure of the single-seed descent
The hard red spring wheat cultivar Duclair was developed by use of the single-seed procedure of single-seed descent [JPR 5:349-352 (2011)]. Some of the information provided below is not in the article and was obtained directly from the senior author.
- Step 1. In the greenhouse, the breeders crossed the cultivar Choteau with the line MT0249 to form a single-cross population.
- Step 2. The F1 plants were grown in the field and F2 seed was harvested.
- Step 3. The F2 seed were planted in the greenhouse in September. One head from each plant was removed. The plants in the greenhouse are small and tillering generally does not occur; therefore, the plants can be distinguished from each other. One head that usually contains four to eight seeds is removed from each plant. One healthy seed from each head is bulked together for planting the next generation. The heads are stored as a reserve in case something destroys the subsequent planting.
- Step 3. The F3 seeds were planted in the greenhouse. Each plant was harvested individually to form an F3:4 line.
- Step 4. The F3:4 lines were planted in Bozeman, MT, during May. Individual lines were selected and single plants were harvested from the selected rows. The authors refer to the F3:4 headrows as F4 head rows. In their system of nomenclature, the row is described by the generation of the plants that are growing and does not describe when the line was derived.
- Step 4. The F4:5 lines (F5 headrows) were planted for evaluation. The F4-derived lines were evaluated in subsequent generations. One of the F4-derived lines became the cultivar Duclair.
Example 3: Multiple-seed procedure of single-seed descent
The breeders of ‘UA 4910’ soybean referred to their method of generation advance as modified single-pod descent [JPR 5:49-53 (2011)]. In the text for this course, their procedure is called the multiple-seed procedure of single-seed descent. When the term “modified” is used for a method of generation advance, it means that the breeders modified what they considered the standard way that a method would be carried out. The only way to understand what was modified is to carefully ready what they did in comparison to what would be the standard procedure.
- Step 1. In 1997, the cross of Asgrow A4715 to DP 3478 was made in the field at Fayettevile, AR, to obtain a single-cross population.
- Step 2. The F1 plants were grown in the 1997-1998 winter nursery in Costa Rica and F2 seed was harvested.
- Step 3. In 1998, the F2 population was grown in the field and two to three pods each containing two or three seeds were harvested from about 600 plants and the pods were threshed in bulk. Part of the seed was planted in Costa Rica (season 2).
- Step 4. The F3 plants were grown in the 1998-199 winter nursery in Costa Rica. Two to three pods each containing two or three seeds were harvested from the plants and threshed in bulk.
- Step 5. The F4 seed was planted in Keiser, AR, during 1999, and 60 plants from the population were harvested and threshed individually. The progeny of the plants were evaluated as F4-derived lines in subsequent generations; therefore, UA 4910 was an F4-derived cultivar.
Example 4: Pedigree method
Georgia-09B peanut cultivar was developed by use of the pedigree method with a backcross population [JPR 4:175-178 (2010)].
- Step 1: The cultivar Georgia Green was crossed to the line GA 942004. The F1 generation was grown to obtain F2 seed. F2 plants were grown and harvested individually. A sample of seed from each F2 plant was evaluated for oleic acid concentration in the oil and one of them was chosen for crossing as a F2:3 line.
- Step 2: In 1998, the F2:3 line was backcrossed to the recurrent parent Georgia Green to obtain BC1F1 seed. The goal of the backcross was to develop a population that had individuals with the desirable agronomic traits of Georgia Green and the high oleic and low linolenic acid traits of the donor parent GA 942004.
- Step 3: The BC1F1 seed was planted at the Univ. of Georgia Coastal Plain Experiment Station and the BC1F2 seed was harvested.
- Step 4: The F2 seed was space planted and individual plants were selected based on plant and seed characteristics. The BC1 designation used by the authors to precede the F designation of every generation will be left off in the following description so that the generation designations will be the same as used for other population types, such as a single cross or three-way cross.
- Step 5: The selected F2 plants were grown as F2:3 lines in two-row plots. Lines with desirable traits were selected and one or more individual F3 plants with desirable traits were selected from within the selected lines.
- Step 6: The selected F3 plants were grown as F3:4 lines in two-row plots and one or more desirable F4 plants were harvested individually from selected lines. The F3:4 lines that traced to single plant selections from the same F2:3 row the previous season would be grown in adjacent rows and referred to as a family. In the standard pedigree method, the breeder would first select the best families. Second, the best F3:4 line(s) within the selected families would be identified. Third, the best individual F4 plants within the selected lines would be harvested and threshed individually.
- Step 7: The selected F4 plants were grown as F4:5 lines in two-row plots. The best lines were selected and threshed in bulk to obtain F4:6 seed. As in the step 6, the breeder would select the best families, followed by selection of the best line(s) within the selected families.
- Step 8: The F4-derived lines were evaluated in replicated tests for three years. Georgia 09B was a F4-derived cultivar.
Example 5: Early-generation testing of selfed lines
The developers of ‘Barlow’ hard red spring wheat described their inbreeding procedure as a combination of the modified bulk and pedigree methods. The process also involved early generation testing of selfed lines [JPR 5:62-67 (2011)]. This example is a good illustration of how breeders use combinations of methods to advance a population.
- Step 1: The cross of the line ND 744 to the line ND 721 was made in the fall greenhouse at North Dakota State University in Fargo, ND.
- Step 2: The F1 seeds of the single-cross population were grown in the greenhouse in the spring of 2000.
- Step 3: The F2 population was planted in the field during the summer of 2000. Spikes were harvested from 200 plants selected for their reaction to leaf rust, plant vigor, height, and earliness. Twenty of the spikes were selected and threshed individually. Selection among individual F2 plants is the first step in the pedigree method.
- Step 4: The 20 F3 headrows (F2:3 lines) were grown in Christchurch, New Zealand, during the winter of 2000-2001. Desirable lines were selected and each line was threshed in bulk to obtain F2:4 seed. In the typical pedigree method, one or more individual F3 plants would have been harvested from the selected lines. The breeders used the term “modified bulk” because they harvested each F2:3 line in bulk, instead of harvesting individual plants.
- Step 5: The selected F2:4 lines were planted in a yield test during the summer of 2001. A spike from 10 individual F4 plants were harvested from the F2:4 lines with desirable yield and other agronomic traits. The evaluation of the F2:4 lines in replicated tests for quantitatively inherited traits represented an early generation test. It is considered an early generation test because the F2:4 lines were not adequately homozygous and homogeneous to merit release as cultivars per se. The purpose of the test was to select lines for quantitative traits based on replicated tests whose progeny had the potential to become cultivars.
- Step 6: The seed from each of the ten spikes was planted in a headrow (F4:5 line) in New Zealand during the winter of 2001-2002. One F4:5 line was selected and threshed in bulk. This F4-derived line was tested in subsequent generations and became the cultivar Barlow. In the standard pedigree method, the 10 F4:5 lines that were derived from the 10 individual spikes of each selected F2:4 line the previous season would be considered a family. The breeder would select the best families, followed by selection of the best line(s) within the best families.
Example 6: Development of lines by the soybean breeding program of Iowa State University.
The following pictures taken by Walter Fehr illustrate one method used by the soybean breeding program at Iowa State University to develop lines for evaluation as potential cultivars.
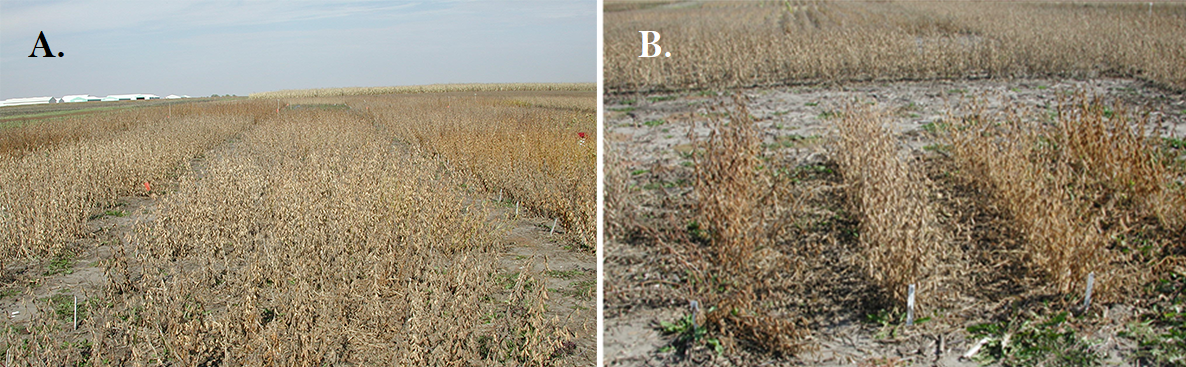
In this example, the crosses are made at Ames during the summer, the F1 seeds are planted near Santa Isabel, Puerto Rico, the middle of October, the F1 plants are harvested in January, the F2 seeds are planted in early February, the F3 seeds are harvested in May by the multiple-seed procedure of single-seed descent, the F3 seeds are planted at Ames in May, and individual F3 plants are harvested individually in the fall to form F3-derived lines for subsequent evaluation.
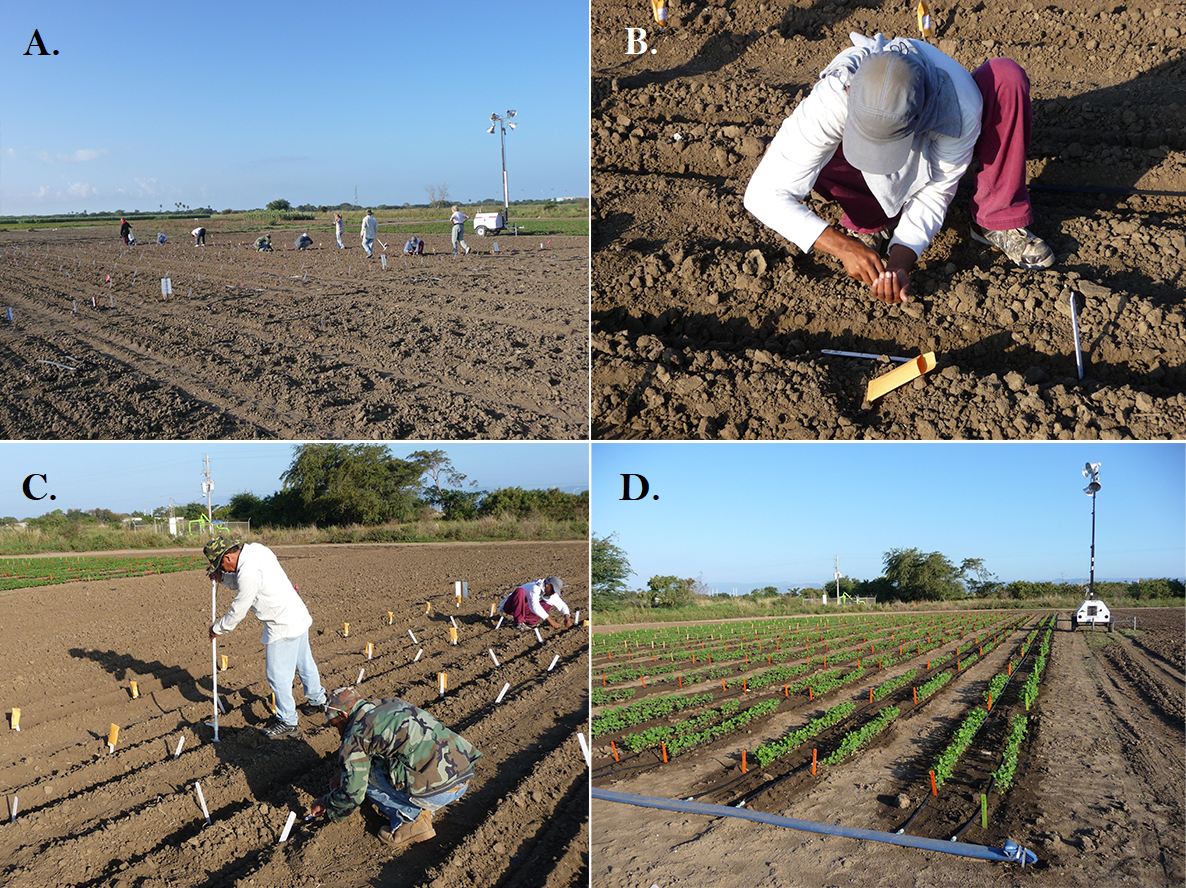
Review Questions 1
In the following questions, assume that S0 = F2.
-
- You are breeding for resistance to a herbicide and to an insect in a diploid species. Resistance to the herbicide is controlled by a single dominant allele (H) at one locus. Insect resistance is controlled by a single recessive allele (i) at a locus independent of the one for herbicide resistance. You cross a parent with the genotype (HHII) to one with the genotype (hhii).
-
-
- Which of the two parents would you use as the female parent in the cross to be able to distinguish phenotypically the hybrid F1 plants from those resulting from accidental self-pollinations? Why is the elimination of accidental self-pollinations important in a breeding program?
- How could you use a molecular marker to distinguish the hybrid F1 plants from those resulting from accidental self-pollinations?
- Under what circumstances would a phenotypic assay for hybrids versus accidental selfs be a better choice than a molecular assay?
- You grow the F2 population and spray it with the herbicide. What would be the genotypic and phenotypic frequencies of the remaining plants for herbicide resistance and for insect resistance?
- You harvest one seed from each of the remaining F2 plants, grow the F3 population, and spray it with the herbicide. What would be the genotypic and phenotypic frequencies of the remaining plants for herbicide resistance and for insect resistance?
- You harvest the F3 plants individually and plant a progeny row of each as a F3:4 line. You spray the lines with herbicide and infect them with the insect. How would you distinguish between homogeneous lines versus heterogeneous lines for herbicide resistance and insect resistance? What would be the expected frequency of F3:4 lines that are homogeneous for herbicide and insect resistance?
-
- You are breeding for modified protein content in a diploid species that is controlled by recessive alleles at three independent loci. Conventional varieties have the genotype G1G1G2G2G3G3. A parent line with modified protein content has the genotype g1g1g2g2g3g3. The wild-type and recessive alleles at each locus differ by a single nucleotide. You cross a conventional variety to the parent line with modified protein content.
-
- You self the F1 plants, grow the F2 population, and test the plants for each of the three loci with the SNP markers. What would be the frequency of F2 plants homozygous for the recessive alleles at all of the loci, g1g1g2g2g3g3?
- Instead of testing the F2 plants, you harvest one seed from each and use the harvested F3 seed to plant the next generation. You harvest one seed from each F3 plant and plant the F4 population. What would be the frequency of F4 plants homozygous for the recessive alleles at all of the loci?
-
Review Questions 2
- You want to develop 100 F4:5 lines of wheat from a single-cross population. Outline season-by-season how you would develop the lines by each of the following methods. Indicate the number of seeds planted and harvested each generation beginning with F2 seeds. The number of seeds planted should be realistic for meeting the goal of obtaining 100 F4:5 lines. Assume that the germination percentage is 80% and that an average of 60 seeds are produced on each plant.
- Single-seed procedure of single-seed descent
- Multiple-seed procedure of single-seed descent
- Bulk method
- Pedigree method
- Explain to your research director what factors you would consider in selecting one of the methods for use for inbreeding your population. Include in your explanation a discussion of the genetic and financial considerations that would be important.
Review Questions 3
The use of doubled haploids is becoming popular for developing inbred lines used in hybrids, including canola and maize. Use the assigned readings and online resources to answer the following questions.
- Outline season-by-season how breeders develop doubled haploids of canola and maize:
- Why has the use of doubled haploids become so popular in the two crops?
- Are there any disadvantages of doubled haploids compared to the use of traditional selfing for development of inbred lines?
The process of mating closely related cultivars, or even self-fertilizing, for several generations to select for higher homozygosity.
The bulk method allows natural selection to act and remove undesirable genotypes from the population.
A breeding method used in the development of both self-pollinated (to develop pure-lines) and cross-pollinated crops (to develop inbreds).
A breeding method used to rapidly advance lines to homozygosity so that selection can be practiced on homozygous lines.