Heterosis
Walter R. Fehr and Walter P. Suza
Readings:
- Chapter 9 [PDF], Principles of Cultivar Development. Vol. 1: Theory and Technique, by Walter R. Fehr (Access the full book)
- Chapter 35 [PDF], Principles of Cultivar Development. Vol. 1: Theory and Technique, by Walter R. Fehr
- Chapter 4, Breeding Vegetable Crops, by Mark J. Bassett (Ed.) (Access online via Internet Archive)
Hybrid cultivars are preferred over pure-line or synthetic cultivars for plant species in which the yield of grain or forage is the primary trait of economic importance. One reason for the interest in hybrid cultivars is that they often yield more than pure-line or synthetic cultivars. The second reason is that it is not practical for a farmer to save seed of a hybrid cultivar to plant the following season because the harvested seed will segregate like an F2 population. As a result, seed companies are assured that the farmer will have to buy new hybrid seed every planting season. In contrast, seed of a pure-line or synthetic cultivar that is harvested one season can be planted the following season.
There are four requirements that must be met to produce a commercially viable hybrid cultivar of a plant species.
Requirement 1: Heterosis
Heterosis, commonly referred to as hybrid vigor, can be expressed in two ways. Mid-parent heterosis is when the performance of the hybrid exceeds the mean performance of its parents. Although this type of heterosis commonly is measured in scientific studies, it is not of commercial interest because one of parents may perform better than the hybrid. High-parent heterosis is when the hybrid performs better than either parent. A plant species must exhibit sufficient high-parent heterosis to be commercially viable.
The genetic and molecular basis of heterosis remain unresolved. There are some scientists that believe it may be a combination of all of the possible causes (See chapter 9 in Principles of Cultivar Development). Their hypothesis is that heterosis for the alleles at some loci may be caused by dominance, for others it may be overdominance, and for others it may involve epistatic interactions.
Heterosis is measured by evaluating the hybrid performance from mating two or more parents. The ability of a parent to mate with others to produce a superior hybrid is referred to as its combining ability. Specific combining ability refers to the ability of a parent to combine specifically with another parent. General combining ability is the average ability of one parent to combine with a group of other parents. These terms will be discussed further in the lessons dealing with hybrid and synthetic cultivars.
For breeding hybrid cultivars, parent germplasm for some species is divided into heterotic groups. Hybrid cultivars generally have greater performance when the inbred or clonal lines developed from one heterotic group are mated to genotypes from another heterotic group than when genotypes from the same heterotic group are mated to each other. Maize is an example of a species in which heterotic groups are important for maximizing the performance of hybrid cultivars. One heterotic group is referred to as the Iowa Stiff Stalk Synthetic, which was developed by corn breeders of the USDA-ARS and Iowa State University. The other heterotic groups are referred to as non-Stiff Stalk. They include the maize populations Lancaster and Reid Yellow Dent. Breeders find that the best hybrid performance generally is obtained by crossing inbreds from the Stiff Stalk Synthetic with those from one of the other heterotic groups.
Heterotic groups sometimes arise as a result of the mechanism used to produce commercial hybrid seed. For example, use of cytoplasmic-genetic male sterility (CMS) involves the use of female inbreds (A-lines) whose genes in the cytoplasm and nucleus result in male sterility. The male inbreds (R-lines) have restorer genes that override the genes in the cytoplasm and result in a male fertile hybrid. Breeders keep separate the germplasm used for developing the two types of lines, which ultimately leads to unique heterotic groups. The same principle applies to the use of self-incompatibility for producing hybrid cultivars of some crops. Separate germplasm pools with different genes for self-incompatibility are maintained, which leads to unique heterotic groups.
Requirement 2: Elimination of fertile pollen from the female parent
Preparation of the female parent for hybridization involves the elimination of fertile pollen to prevent self-pollination. Table 8.1 lists methods commonly used in hybrid vegetable seed production.
Method/mechanism used | Examples of crops |
---|---|
Hand emasculation plus hand pollination | Tomato, eggplant, sweet pepper, okra, hot pepper |
Pinching of staminate flowers plus hand pollination | Curcubits (bitter gourd, bottlegourd) |
Male sterility plus hand pollination | Tomato, hot pepper, sweet pepper |
Male sterility plus natural pollination | Onion, cabbage, cauliflower, carrot, radish, muskmelon, hot pepper |
Self-incompatibility plus natural pollination | Most of the cole vegetables like broccoli, cabbage |
Gynoecism plus natural pollination | Cucumber, muskmelon |
Pinching of staminate flowers* plus natural pollination | Curcubits (bitter gourd, summer squash) |
Plant growth regulator of staminate flowers* plus natural pollination | Summer squash, winter squash |
*Genotypes with increased proportions of pistillate flowers are desirable for hybrid development.
Manual emasculation
In some species, the elimination of fertile pollen from the female parent of a hybrid is accomplished by hand emasculation of perfect flowers or manual removal of staminate flowers from flowers from monoecious plants (Kumar and Singh, 2004). Tomato will be used to illustrate the principles associated with the use of manual emasculation in a species with perfect flowers. An excellent description of the manual process used to obtain commercial hybrid seed of tomato was developed by Ope a, Chen, Kalb, & Hanson (2001) [PDF].
There are some important requirements for the practical use of manual emasculation for species with perfect flowers. (1) The cost of hand labor must be low enough so that the cost of the hybrid seed is acceptable to the consumer. Consequently, the production of hybrid seed by manual labor generally is done in countries where labor is less expensive than in the United States. (2) The number of hybrid seed from a single pollination should be high. In tomato, the number of hybrid seeds from a single pollination (fruit) is about 200. (3) The value of a hybrid plant must be sufficiently greater for the end user than that of a pure-line cultivar.
Maize is an example of a monoecious species for which manual removal of the staminate flowers (detasseling) for commercial hybrid seed production is commonly practiced. Manual emasculation also can be practical in plant species with monoecious flowers (Kumar and Singh, 2004). A description of the detasseling process can be found in this detasseling video (https://youtu.be/W3Ar4sdL-JI).
Genetic (nuclear) male sterility
Major nuclear genes have been found in many plant species that cause the pollen to be inviable, the most common of which are recessive. The primary use of the genes for hybrid seed production has been in vegetable crops in which they provide an alternative to hand emasculation, including tomato, pepper, and okra. The problem with use of genetic male sterility is that the female parent of the hybrid segregates for male-fertile and male-sterile plants. Plants of the female parent must be inspected individually and the male-fertile ones removed before pollination, which is labor intensive and expensive process. These costs are only acceptable for producing seed of species for which hybrid plants are valuable enough to justify the expense.
Pepper will be used to illustrate the principles involved with the use of genetic male sterility (Hundal and Dhall, 2004). Genetic male sterility in pepper, as in many other species, is controlled by the recessive allele ms. One method that can be used to develop the inbred line used as the female parent for hybrid seed production begins with selecting male fertile F2 plants (MsMs or Msms) in a population segregating for the ms allele, as described in Chapter 16 of the text. The Msms plants are identified by progeny testing the F2:3 lines and selecting those segregating for male-fertile and male-sterile plants. Within the segregating rows, multiple individual male-fertile F3 plants are harvested and progeny tested as F3:4 lines to identify those that are segregating. Male-fertile F4 plants are harvested within the segregating line and progeny tested as in the previous generations. The process is continued until the lines are considered adequately homozygous. If a line is selected for use in a hybrid, seed is harvested from its male-sterile plants that have been pollinated by male-fertile plants in the same line. The seed from the male-sterile plant will be male-sterile (msms) and male-fertile (Msms). In subsequent generations of seed production, the only male-fertile plants present will be Msms and the resulting seed used to plant the female parent for hybrid seed production will segregate in a ratio of 50% male sterile and 50% male fertile. The male-fertile plants are removed by hand before pollination. The remaining male-sterile plants are pollinated by the male-fertile male parent (MsMs). The male parent can be developed by use of conventional inbreeding methods.
An alternative method for developing the female inbred is to first identify an elite male-fertile (MsMs) line, followed by backcrossing the ms allele into it. This would eliminate the need for identifying Msms individuals within a line during inbreeding, which would simplify the inbreeding process. However, it would take additional time to carry out the backcrossing program after the elite line was identified.
The ideal situation for eliminating the male-fertile plants in the female parent would be to have a gene tightly linked to the Ms allele that produces a plant phenotype that can be readily observed before flowering. Plants with that phenotype would be discarded as male-fertile individuals. Such linkages have been proposed for many years, but none have been used in hybrid seed production .
Gynoecious inbreds
Gynoecious inbreds that have only pistillate flowers are used as the female parent for hybrid seed production in some monoecious and dioecious species. This method of eliminating male pollen from the female parent will be illustrated with asparagus, a dioecious species, that has genes on X and Y chromosomes that control sex expression and with cucumber, a monoecious species, for which the genes controlling sex expression are not associated with X and Y chromosomes.
Asparagus
Asparagus is a dioecious species with X and Y chromosomes that determine whether a plant has female or male flowers (Ellison, 1986; Anido and Cointry, 2008). Female plants have XX chromosomes, males have XY, and supermales have YY. Most commercial hybrids are produced by mating a gynoecious female parent (XX) to an androecious supermale inbred (YY) to obtain a androecious male hybrid (XY). Male hybrids yield more and live longer than females. The primary commercial method of vegetatively propagating the male hybrid is with plants derived from meristem tissue culture. For home use, male hybrids can be propagated by pieces of the rhizome (crown).
According to Dr. Chin, the asparagus breeder at Rutgers University (http://aesop.rutgers.edu/~asparagus/research/PROGRAM.HTML), the primary methods currently used for obtaining inbred lines is by self-pollination of andromonecious (XY) (Figure 1) and anther culture.
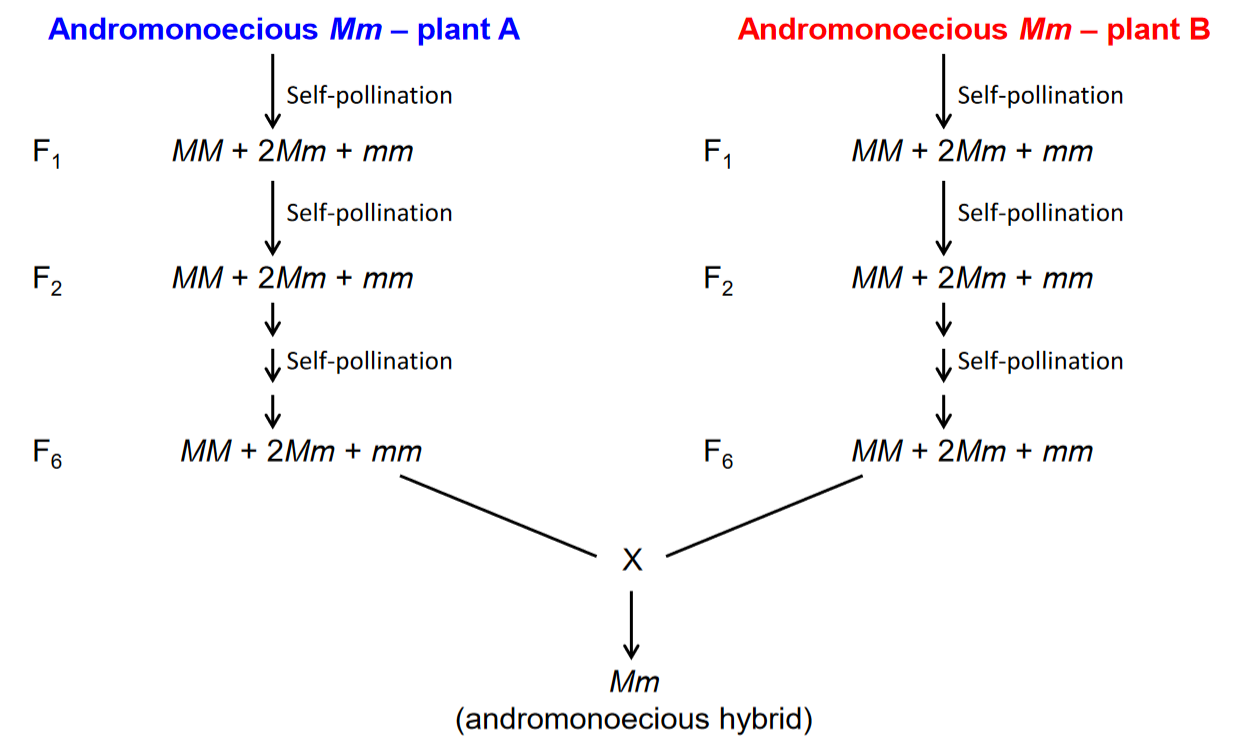
An andromonoecious plant produces enough hermaphroditic (perfect flowers) for self-pollination. After the breeder considers a line to be adequately homozygous, the gynoecious females (XX) or the supermales (YY) in the selfed progeny are selected. According to Dr. Chin, the best hybrids commonly are obtained by crossing a female from one location of origin to a supermale from another location of origin.
For anther culture, anthers from male (XY) plants in the population are used to generate homozygous female (XX) and homozygous supermales (YY). Although the frequency of such individuals is low, fewer years are required to generate potential inbreds than by self-pollination.
Cucumber
There are three major genes that control the flower type and distribution for cucumber. The gene symbols used for the three genes differ among authors, such as that used by Lower and Edwards (1986) in Breeding Vegetable Crops. In this lesson, the symbols M,m; F,f; and A,a will be used as reported by Call and Wehner (2010) and Staub et al. (2008).
The three flowering types of practical importance for producing hybrids are gynoecious (MMFF), monoecious (MMff), hermaphroditic (mmFF), and andromonoecious (mmff). The A,a alleles do not influence these four flowering types. The androecious type has the genotype ffaa and is not influenced by the M,a allele. The andromonoecious and androecious types generally are not involved in breeding or production of commercial hybrids.
Several types of hybrids are used commercially in cucumber. Gynoecious hybrids are preferred for commercial-scale production because they flower earlier than monecious hybrids and all of the fruit can be harvested mechanically at one time, instead of multiple harvests with monoecious hybrids. Monoecious hybrids are used for home gardens. A description of seed production of these hybrid types can be found at Call and Wehner (2010). Information on field production of cucumber can be found at Valenzuela, Hamasaki, and Fukuda (n.d.).
Gynoecious inbred (MMFF) x Gynoecious inbred (MMFF) = Gynoecious hybrid (MMFF). For the production of hybrid seed, silver nitrate or silver thiosulfate are sprayed on the male parent to inhibit formation of the plant hormone ethylene, which stimulates the production of staminate flowers. About 45% of cucumber acreage in the USA is of this hybrid type (Staub, personal communication, 2010). For field production, about 15% of monoecious hybrid seeds that serve as pollinators are blended with the gynoecious hybrid seed before planting (Staub et al., 2008). Gynoecious x gynoecious hybrids commonly are part of the system used for parthenocarpic production of seedless cucumbers in the greenhouse. Parthenocarpic cucumbers are formed without pollination. The parthenocarpic trait is under separate genetic control than the M and F genes that control the gynoecious trait (De Ponti, 1976). Therefore, the breeding of gynoecious lines for parthenocarpic production is more complex than breeding gynoecious lines for use in hybrids whose fruit is produced through pollination in the field.
The selfing of gynoecious plants for inbreeding is accomplished by treating them with silver nitrate or silver thiosulphate to stimulate the production of staminate flowers. The methods used for generation advancement are discussed further in Plant Breeding Methods. Use of the methods in cucumber is described by Staub et al. (2008).
Gynoecious inbred (MMFF) x Monoecious hybrid (MMff) = Gynoecious hybrid (MMFf). This hybrid type is common used for large-scale commercial in the field. About 15% of monoecious hybrid seeds that serve as pollinators are blended with the gynoecious hybrid seed before planting.
The selfing of monoecious plants for inbred line development can be readily accomplished because there are pistillate and staminate flowers on the same plant.
Gynoecious inbred (MMFF) x Hermaphroditic inbred (mmFF) = Gynoecious hybrid (MmFF). About 15% of monoecious hybrid seeds that serve as pollinators are blended with the gynoecious hybrid seed before planting. This hybrid type is not used in the United States because the shape of the fruit produced by the hybrid is not usually acceptable.
Monoecious inbred (MMff) x monoecious (MMff) inbred = Monoecious hybrid (MMff). For production of hybrid seed, the growth regulator ethephon (brand name Ethrel) is applied to the female parent to increase the production of ethylene, which results in the formation of pistillate instead of staminate flowers. This hybrid type is desirable for home use because flowering and fruit production extend over a longer period of time than for gynoecious hybrids. It also is used in countries such as Mexico for long-season production of cucumber (Todd Wehner, North Carolina State Univ., personal communication, 2010)
Hermaphroditic inbred (mmFF) x hermaphroditic (mmFF) inbred = Hermaphroditic hybrid (mmFF). This type of hybrid is not grown in the United States because the unattractive spherical fruit with big blossom-end scars is not acceptable to consumers (Jack Staub, personal communication, 2010).
Self-imcompatibility (SI)
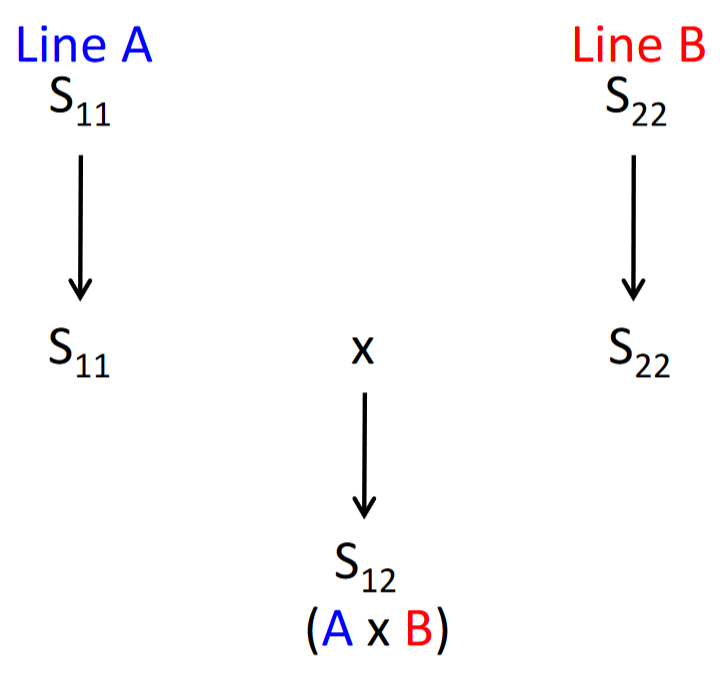
Production of hybrid seed of some vegetable crops is carried out through the use of SI genes, including cabbage, broccoli, kohl rabi, and cauliflower. One parent of the hybrid has one set of SI genes and the other parent as another set (Figure 2).
The principles of the SI system of any crop are described in detail by Kalia and Sharma (2004) with broccoli. The primary method used to overcome SI for inbreeding is bud pollination. Bud pollination involves the application of pollen from a plant on the stigma of a flower of the same plant before the SI mechanism is activated.
One limitation of the SI system for hybrid seed production is the production of enough self-pollinated seed of each parent for planting a hybrid seed production field. Another limitation of the system is obtaining inbred lines with stable SI so that self-pollinated seed is not produced during hybrid seed production. These problems with the SI system have restricted its use to certain vegetable species for which the costs of implementing the system can be covered by the value of the hybrid seed.
Cytoplasmic-genetic male sterility
The cytoplasmic-genetic male sterility (CMS) system makes it possible to obtain female plants in a hybrid seed production field without the roguing required when genetic male sterility is used alone. CMS is used extensively in species with perfect flowers and in some species with monoecious flowers. The system involves an A-line that is the male-sterile female parent used in the hybrid seed production field, the B-line is the maintainer used to produce seed of the A-line, and the R-line (restorer) is the male parent used in the hybrid seed production field.
The unique aspect of the CMS system is the use of a cytoplasm that renders the plant male sterile, unless the nucleus has genetic factors in the nucleus that overcome the cytoplasmic factors and restore male fertility. The cytoplasm of an individual is transmitted to its seed through the egg cell and not through the pollen.
To develop an elite A-line for the CMS system, it is impossible to cross an A-line to another A-line to develop a segregating population because both parents would be male sterile and no seed would be produced. Therefore, the breeder first must develop a superior male-fertile B-line with normal cytoplasm and non-restorer (rf) genes by crossing two or more B-line parents together to obtain a segregating population. The details of how to select a superior B-line from the population is the topic of Lesson 9. A new elite B-line is used as the recurrent parent in a backcross to develop an A-line that is as genetically identical as possible to itself for all the nuclear genes. Both the B-line and A-line would have non-restorer genes; however, the A-line would be male-sterile because it has the factor in its cytoplasm that renders its pollen male sterile.
A new elite R-line must have dominant nuclear genes (Rf genes) that overcome the sterile cytoplasm of the A-line, resulting in a male-fertile hybrid for the farmer. To develop a segregating population for selection, R-line parents are crossed to each other to assure that individuals in the population will have the necessary restorer genes. After an elite R-line is identified, it can be used directly as the male parent for hybrid seed production.
Seed of the A line is produced by crossing it to its B-line maintainer. For a crop such as maize, the A-line and B-line are planted in strips similar to that illustrated in figure 7. The row of the B-line is destroyed after pollination to avoid accidentally harvesting it. Seed of the B-line and R-line are produced by planting each of them in isolation and letting them naturally pollinate.
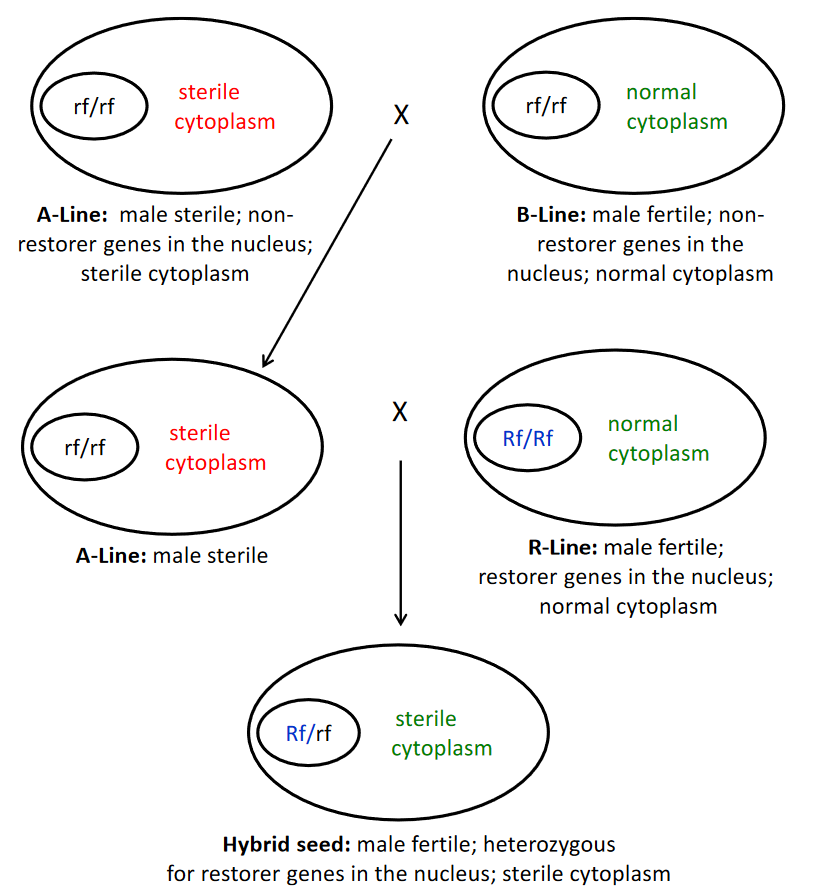
Transgenic methods for the development of male sterile parents
The SeedLink Invigor system
A gene named barnase from the soil bacterium called Bacillus amyloliquefaciens makes a defense protein that destroys RNA molecules to kill potential enemies. The bacterium also produces another protein called barstar that it uses to protect itself from the toxicity of barnase [Nature. 347:737-741 (1990)]; [Nature. 357:384-387 (1992].
Scientists at Bayer CropScience developed the SeedLink Invigor system for canola hybrid seed production that utilizes the barnase (BS) and barstar (BR) genes linked with the bar (L) gene for glufosinate (Liberty) tolerance. More information about the genetic elements used in the SeedLink Invigor system can be found on pages 9, 11 and 12 of Bayer CropScience application for deregulation of the system in Europe: MS8/RF3 Request for Renewal [PDF]. The principle components of the system are illustrated in figure 2, and explained in greater detail in the figure below. .
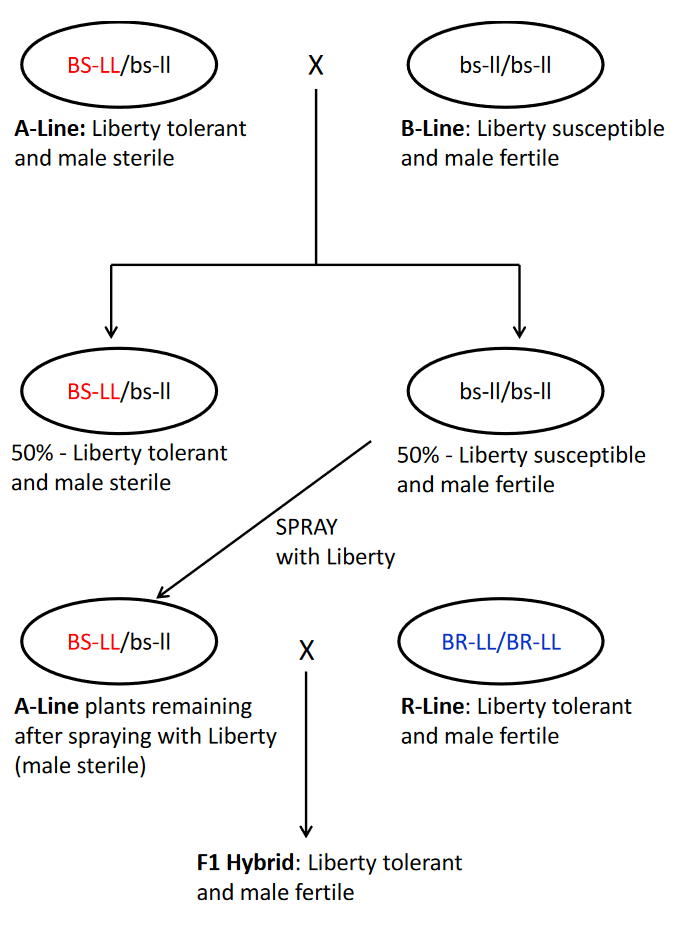
The following is an explanation of figure 4 and describes how the SeedLink Invigor system is implemented by a canola breeder.
Development of the A- and B-lines
Step 1. The breeder develops new B-lines from breeding populations formed by crossing two or more B-line parents to each other, the same as for the CMS and SPT systems. The populations and elite B-lines derived from them lack the transgenes associated with the Invigor system.
Step 2. An A line is developed that is genetically identical to the B-line, but that has the BS-LL transgene. This is accomplished by crossing an elite B-line to plants of an available A-line that are heterozygous for the transgene. Because the A-line is heterozygous, 50% of the F1 plants from the cross will be heterozygous for the transgene and 50% will be homozygous for absence of the transgene. The F1 plants are sprayed with Liberty herbicide to kill those that lack the transgene. The heterozygous male-sterile F1 plants are backcrossed to the B-line that serves as the recurrent parent. The BC1F1 plants will segregate for the transgene in the same ratio as the F1 plants. When they are sprayed with Liberty, the surviving heterozygous male-sterile BC1F1 plants will be crossed to the B-line to produce BC2F1 seed. This process will continue for as many backcrosses as desired to obtain an A-line and its B-line maintainer that are genetically the same, except for the BS-LL transgene.
To produce seed of the A-line for planting a hybrid seed production field, the A-line is planted in alternating strips with its B- line. The A-line will segregate with 50% of the plants containing the BS-LL transgene and 50% lacking the transgene. The A-line strips are sprayed up to three times before pollination with glufosinate herbicide to kill the plants lacking the BS-LL transgene. At maturity, the A-line strips are harvested.
To obtain seed of the B-line that is needed for producing seed of the A-line, it is planted in an isolated field that is not sprayed with glufosinate.
The R-lines used as the male parent for hybrid seed production can be developed from populations lacking the BR-LL transgene or from populations in which one or both parents have the transgene. If the elite R-line lacks the transgene, it must be incorporated by backcrossing. When backcrossing is completed, seed of the R-line is produced in an isolated field for use in a hybrid seed production.
The hybrid seed production field will have strips of the A-line and R-line. The A-line will be sprayed at least three times with glufosinate to kill the 50% of the plants that lack the BS-LL transgene and are male-fertile. The remaining plants are heterozygous for the transgene and male-sterile. The R-line is homogeneous for the BR-LL transgene and male-fertile. None of the plants in the R-line will die if sprayed with Liberty. The seed harvested from the A-line is the hybrid seed that will be sold to the farmer. The hybrid plants grown by the farmer will have the genotype BR-LL/br-LL and BS-LL/bs-LL, which makes them tolerant of glufosinate and male-fertile because the BR gene is dominant to the BS gene.
The Seed Production Technology (SPT) system
The SPT system of Dupont/Pioneer is an alternative to the CMS system for producing hybrid seed corn without detasseling. One weakness of the CMS system in corn is the possibility that the cytoplasmic factors that cause male sterility could make the hybrid plants in a farmer’s field susceptible to a disease, as occurred in the 1970’s with the Southern corn leaf blight.
The SPT system involves a transgene the DsRed2 (Alt1) gene, designated “F” in this description, that produces a fluorescent pinkish-red color in the seed; the wild-type nuclear male-fertility Ms45 gene, designated “Ms”, required for the production of fertile pollen; and the zm-aa1 gene, designated “A”, which produces the α-amylase enzyme that destroys pollen by breaking down starch in the pollen, but does not reduce the viability of egg cells. A description of the system can be downloaded for review: Petition for the Determination of Nonregulated Status for Maize 32138 SPT Maintainer [PDF].
The following discussion explains how the system is implemented by a corn breeder who is responsible for developing new elite inbred lines.
Development of the A- and B-lines
Step 1. The breeder develops elite B-lines from breeding populations formed by crossing two or more B-lines to each other, the same as for the CMS and InVigor systems. The populations and elite B-lines derived from them lack the transgene associated with the SPT system and have the dominant Ms allele for male fertility that is present in essentially all of the corn germplasm used by breeders for inbred line development.
Step 2. An elite B-line that lacks the transgene and has the wild-type Ms allele will have the genotype f-ms-a/f-ms-a Ms/Ms. The f-ms-a/f-ms-a indicates that the transgene is absent. The B-line must be converted into two genetic types by backcrossing: an A-line and a B-line maintainer. The process begins by crossing the new B-line as male to an existing B-line maintainer that is hemizygous for the transgene and homozygous for the recessive ms allele (F-Ms-A/ f-ms-a ms/ms). The recessive ms gene that causes male sterility in the homozygous condition was found in the maize germplasm collection and is not a transgene. The transgene and ms gene are independently inherited and both can be detected in a plant by the use of perfect molecular markers for each. The F1 seed from the cross will have the genotypes F-Ms-A/f-ms-a Ms/ms or f-ms-a/f-ms-a Ms/ms, both of which will be male fertile because they have the dominant Ms allele. The seeds with the genotype F-Ms-A/ f-ms-a Ms/ms are selected based on their fluorescent red color. The F1 plants from the selected seeds are backcrossed to the elite B-line to obtain the BC1F1 seeds, which have the genotype F-Ms-A/f-ms-a Ms/Ms, F-Ms-A/f-ms-a Ms/ms, f-ms-a/f-ms-a Ms/Ms, or f-ms-a/f-ms-a Ms/ms. The seeds with the genotype F-Ms-A/ f-ms-a Ms/ms are selected based on their fluorescent red color and by the use of a molecular marker that can identify heterozygous Ms/ms seeds. In each subsequent backcross generation, the selected seeds are planted and the BCxF1 plants are backcrossed to the elite B-line. When a sufficient number of backcrosses have been made, the BCxF1 plants with the genotype F-Ms-A/- Ms/ms are self-pollinated to recover F2 seeds with the genotype F-Ms-A/ f-ms-a ms/ms to use as the B-line maintainer and F2 seeds with the genotype f-ms-a/f-ms-a ms/ms to use as the A-line. Because the A-line and B-line are derived from the same BCxF1 plants, they will be genetically identical for all of the nuclear genes, except for the transgene.
Development of the R-lines
The R-lines are developed from breeding populations formed by crossing one or more R-lines to each other. The populations and the R-lines selected from them will have the wild-type Ms allele that is in a conventional corn germplasm. The new R-line can be used as is for hybrid seed production without additional breeding.
Production of A-line seed
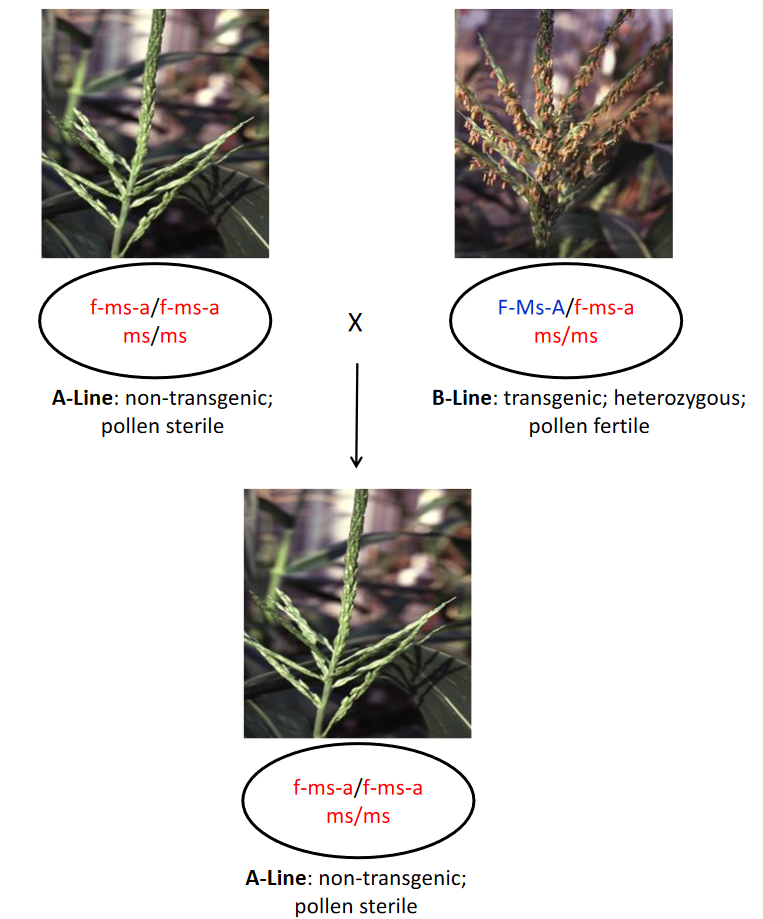
Production of B-line seed
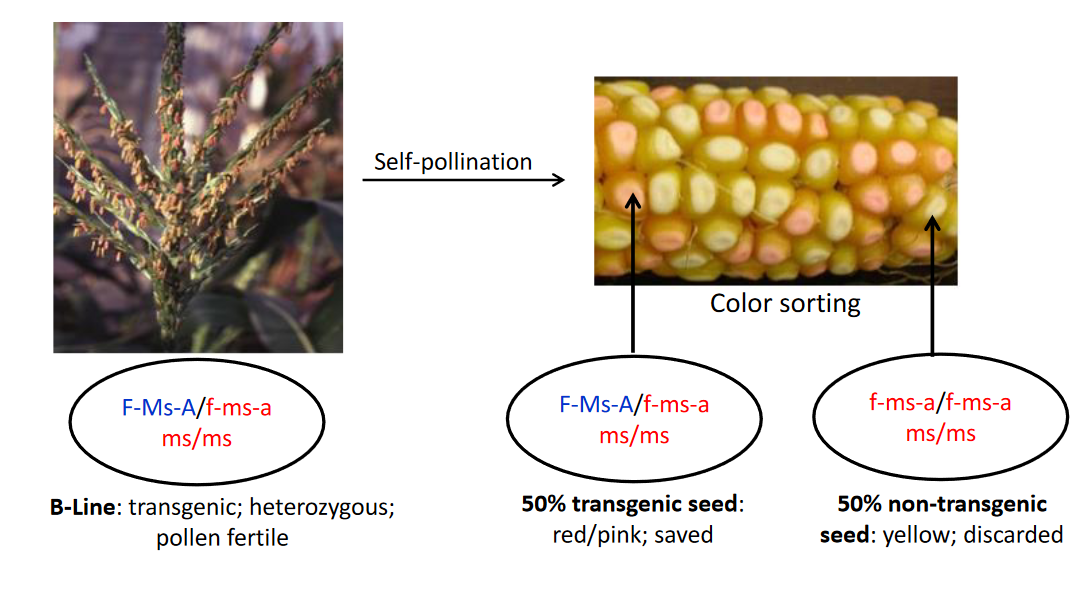
Production of R-line seed
R-line seed is produced by planting it in isolation and letting it naturally pollinate. All of the seed will lack the transgene and be homozygous for the wild-type Ms allele, which makes the R-line male fertile.
Production of hybrid seed
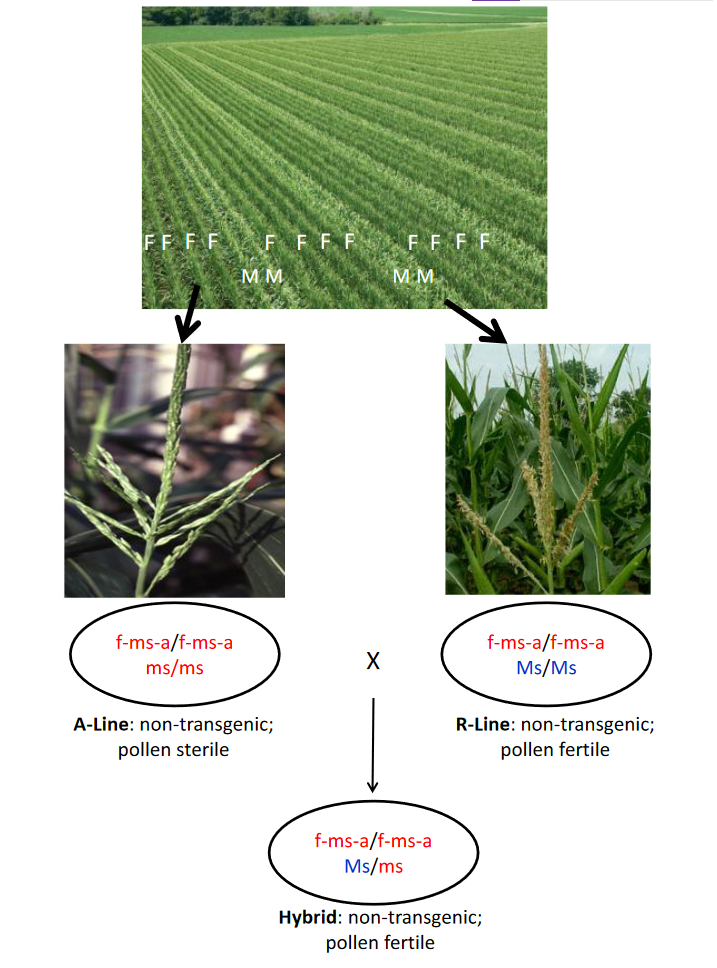
Chemical hybridizing agents (CHA)
The use of CHA to obtain male-sterility of the female parent in a hybrid seed production system has the potential advantage of eliminating the need for a breeder to incorporate specific genetic factors for male-sterility into inbred lines. They have been extensively studied in wheat in comparison with the use of CMS. The studies of Cisar and Cooper (2002) and Adugna (2005) are just two of many that have been published on the subject. Three of the most important attributes of a CHA are that they should cause male sterility without reducing female fertility, the male sterility should be complete, and it should be effective on a range of genotypes. A few CHA have been found that meet most of these attributes and have been used to produce commercial hybrid seed of a few crops that have had difficulty finding reliable CMS systems, such as wheat (Cisar and Cooper, 2002).
Requirement 3: Transfer of pollen from the male to the female parent
The most cost-effective method of transferring pollen from the male to the female parent is by wind or insects. When insect pollination is involved, insects commonly are brought to a commercial field to assure that an adequate number are present, such as in alfalfa, sunflower and canola.
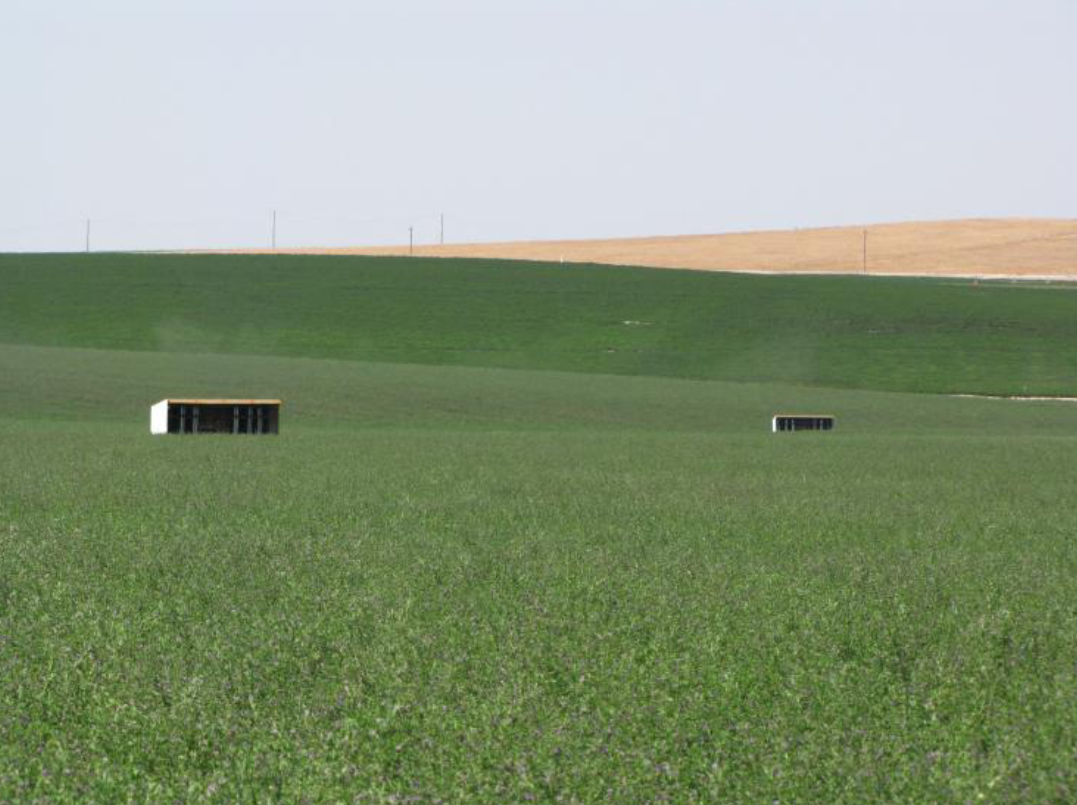
Manual pollination for the production of hybrid seed is done for some species with perfect flowers, such as tomato.
Effective pollen transfer has prevented the use of hybrid cultivars in some self-pollinated species, such as soybean. Although some pollen transfer occurs by insects, the amount of hybrid seed production is too low and unreliable for large-scale production. In wheat, the range of seed set through wind pollination ranges from 10 to 70%, which has limited the use of hybrid cultivars for commercial production (Adugna, 2005).
Requirement 4: Reliable and economical hybrid seed production
The ultimate requirement for hybrid cultivars is that they be profitable for the breeder, the company producing the commercial seed, and the end users. In order for this to occur, the hybrid cultivar must perform better than the other cultivar types of the species to offset the greater seed cost. The greater seed costs are associated with more expense for breeding the parents of the hybrid and the greater cost of producing the hybrid seed.
Applied Learning Activity 8
Breeding research has been done for at least 50 years to develop hybrid cultivars of wheat, yet the area planted to hybrid cultivars is far less than that of pure-line cultivars. Your assignment is to use resources available through the internet to write a report that addresses the following questions. The report should be not more than three pages long, single spaced, with a 12-point font. Cite the references used for the information in the same style as used for research articles in Crop Science.
- How much high-parent heterosis is there in wheat?
- For the hybrid cultivars that have been grown commercially, what methods have been used to eliminate fertile pollen from the female parent?
- How has pollination been carried out to obtain adequate seed set on the female parent?
- What have been the primary factors limiting the widespread use of hybrid wheat cultivars?
References
Andugna, A. 2005. Behavior of bread wheat (Triticum aestivum L.) male sterilized with cytoplasmic-genetic and chemical systems and their impact in hybrid seed production. Asian J. Plant Sci. 4:316-322.
Anido, F. L., E. Cointry. 2008. Asparagus. In: Prohens J, Nuez F (eds). Vegetables II – Fabaceae, Liliaceae, Solanaceae, and Umbelliferae. Springer, New York, NY, pp 87-119.
Call and Wehner, T. 2010. Cucurbit Breeding at NC State. Cucurbit Breeding. http://cuke.hort.ncsu.edu/cgc/cgcgenes/gene10cuke.html
Cisar, G., D. B. Cooper. 2002. Hybrid wheat. In: Curtis B. C., Rajaram
S, Macpherson, G. H. (eds) Bread wheat: improvement and production. Food and Agriculture Organization, Rome, Italy, Available at – http://www.fao.org/docrep/006/y4011e/y4011e00.htm
De Ponti, O.M.B., Garretsen, F. 1976. Inheritance of parthenocarpy in pickling cucumbers (Cucumis sativus L.) and linkage with other characters. Euphytica 25, 633–642. https://doi.org/10.1007/BF00041600
Ellison, J. H. 1986. Asparagus breeding. In: Bassett, M. J. (ed). Breeding Vegetable Crops. AVI, Westport. pp 521-569.
Fehr, W. R. (ed). 1987. Principles of Cultivar Development. Vol 1. Theory and Technique. McGraw-Hill, Inc., New York.
Hundal, J. S., R. K. Dhall. 2004. Breeding for hybrid hot pepper. In: Singh, P. K., Dasgupta, S. K., Tripathi, S. K. (eds). Hybrid vegetable development. Food Products Press, Binghamton, NY, pp 31-50
Kalia, P., S. R. Sharma. 2004. Current researches in hybrid broccoli. In: Singh P. K., Dasgupta S. K., Tripathi S. K. (eds). Hybrid vegetable development. Food Products Press, Binghamton, NY, pp 109-134
Kumar, S., P. K. Singh. 2004. Mechanisms for hybrid development in vegetables. In: Singh, P. K., Dasgupta S. K., Tripathi, S. K. (eds). Hybrid vegetable development. Food Products Press, Binghamton, NY, pp 383-409
Lower, R. L., M. D. Edwards. 1986. Cucumber breeding. In: Bassett, M.J. (ed). Breeding Vegetable Crops. AVI, Westport. pp 173-207.
Mariani, C., M. de Beuckeeler, J. Truettner, J. Leemans, R. B. Goldberg. 1990. Induction of male sterility in plants by a chimaeric ribonuclease gene. Nature 347:737-741.
Mariani, C., V. Gossele, M. de Beuckeeler, M. de Block, R. B. Goldberg, W. de Greef, J. Leemans. 1992. A chimaeric ribonuclease-inhibitor gene restores fertility to male sterile plants. Nature 357:384-387.
Opeña, R.T., Chen, J.T., Kalb, T. and Hanson, P. 2001. Hybrid Seed Production in Tomato. International Cooperators’ Guide. AVRDC. http://web.archive.org/web/20091229013348/http://www.avrdc.org/LC/tomato/seedhybrid.pdf
Staub, J. E., M. D. Robbins, T. C. Wehner. 2008. Cucumber. In: Prohens, J., Nuez, F. (eds). Vegetables I – Asteraceae, Brassicaceae, Chenopodicaceae, and Cucurbitaceae. Springer, New York, NY, pp 241-282.
Valenzuela, H., Hamasaki, R.T., and Fukuda, S. n.d. Field Cucumber Production Guidelines in Hawaii. Crop Production Guidelines. http://www.extento.hawaii.edu/kbase/reports/cucumber_prod.htm
Verna, T. S., S. C. Sharma. 2004. An overview of hybrid khol rabi breeding. In: Singh, P. K., Dasgupta, S. K., Tripathi, S. K. (eds). Hybrid vegetable development. Food Products Press, Binghamton, NY, pp 135-150