Chapter 1: Basic Principles of Plant Breeding
Arti Singh; Jessica Barb; Asheesh Singh; and Anthony A. Mahama
Africa produces a diversity of crops including cereal, pulse, oilseed, root, and tuber species (Table 1), but contributes less than a quarter of the world production of root and tuber crops (Ngopya, 2003). In East Africa, bananas (especially cooking bananas) are an extremely important crop. In Uganda, for example, bananas serve as the largest only source of calories. Even though crop production is mostly for subsistence needs, there is tremendous business potential in local and international markets.
Cereal | Pulse | Oilseed | Root and Tuber |
---|---|---|---|
Maize | Dry beans | Seed cotton | Cassava |
Sorghum | Groundnut | Sesame | Yams |
Millet | Cowpeas | Palm | Sweet Potatoes |
Rice | Soybean | n/a | Potatoes |
Wheat | Cocoa beans | n/a | n/a |
Barley | n/a | n/a | n/a |
- Provide an overview of major crops and production challenges in Africa
- Review or introduce basic breeding principles
- Understand the concepts of setting plant breeding objectives in a program
- Review plant reproductive systems
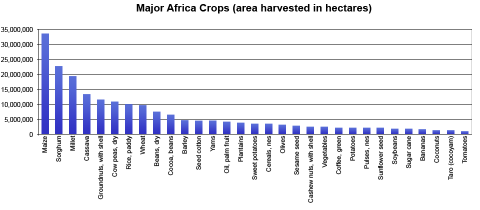
Production Challenges for African Crops
Production of African crops is dependent on yield potential and on protection of yield (that is, realization of yield potential). Numerous factors which reduce production include:
- Biotic constraints to production (i.e., living biological organisms such as disease causing organisms and insects)
- Abiotic constraints to production (i.e., non-living factors such as heat, drought, poor soil fertility)
- Lack of incentive to increase production due to poor marketing opportunities, the lack of infrastructure (such as roads, storage facilities, etc.), and policies that fail to encourage increased production.
- Lack of capital to invest in increasing production
Breeding efforts only address the first two constraints of biotic factors and abiotic factors. The ability to store a crop after harvest is also affected by biotic and abiotic factors. In order to improve food security and provide income to farmers, continuous efforts by plant breeders are needed to increase production per unit area of land while maintaining crop quality.
Systems of Reproduction in Crops
Asexual Reproduction
Plant reproductive systems (or mating systems) fall into three main categories: asexual, autogamous (self-fertilizing), and allogamous (cross-fertilizing). These topics are covered in greater detail in Crop Genetics on Reproduction in Crop Plants.
Asexual reproduction generates individuals that are genetically identical to the mother parent plant and are referred to as clones. The two main forms of asexual reproduction/propagation are vegetative and apomictic. Vegetative propagation is the creation of clones from stem cuttings, suckers (similar to tillers), tubers, runners (stolons), rhizomes, bulbs, scions, and other plant parts. Cassava, sweet potato, and sugarcane are propagated via stem cuttings. Bananas are typically propagated by suckers, while potatoes are propagated by tubers. Elephant grass (Napier grass, Pennisetum purpureum) is propagated by rhizomes, sets (suckers), and stem cuttings. Apomictic reproduction is the asexual propagation of a plant via clonal seeds formed by one of several means that either bypass meiosis or result in a failure of meiosis. Examples of apomictic crops include Citrus and many perennial forage species.
Sexual Reproduction (Self- and Cross-Fertilization)
Sexual reproduction involves the union of a male sperm with a female egg cell or ovary. This process is called fertilization. There are two types of pollination: self-pollination and cross-pollination. When the pollen of a plant pollinates a flower on the same plant the process is called self-pollination (Fig. 2A). Pollination is the transfer of male sperm carried in pollen to the female part of a flower called the stigma (Fig. 2B). When the pollen of a plant pollinates a flower on another plant of the same species the process is called cross-pollination. In nature, cross-pollination requires wind, water, insects, birds, or other animals to transfer the pollen. Most cultivars (i.e., cultivated varieties) are created by a process that involves at least one generation of cross-pollination by plant breeders; including both self- and cross-pollinated species. (See Reproduction in Crop Genetics).
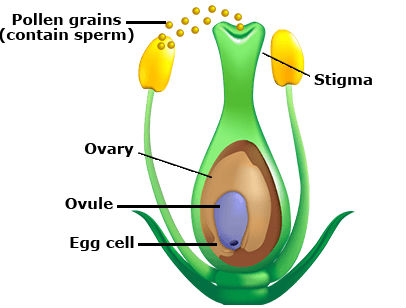
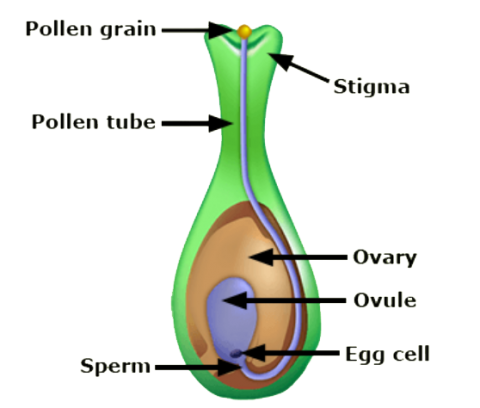
Both the sperm and the egg are haploid, meaning they contain a single set of chromosomes from the male or female parent, respectively. Fertilization unites the single set of chromosomes in the sperm nucleus with the single set of chromosomes in the egg nucleus to produce a complete pair of chromosomes (diploid) in the zygote. Several crop species, for example, banana, sweet potato, potato, and many grasses have three or more sets of chromosomes and therefore they are, in general, called polyploids (e.g., triploids, tetraploids, pentaploids, hexaploids, octaploids).
Flowering plants have a unique process called double-fertilization in which the embryo and the endosperm are fertilized separately. Each pollen grain contains two pollen nuclei; one pollen nucleus fuses with the egg cell to form a diploid zygote and the second pollen nucleus fuses with the two polar nuclei in the ovule, eventually developing into a triploid endosperm. The zygote begins to divide by mitosis forming a multicellular embryo within the ovule. The endosperm provides the energy source that is used by the embryo prior to formation of true leaves that begin photosynthesis. Following fertilization the ovule (with embryo and endosperm) develops into a seed.
Genetic Information Transfer
Genetic information (i.e., DNA) from both the male and female parents is present in a seed produced by fertilization. It is this union of sperm and egg cell that results in the creation of genetic variation (if the male and female gametes possess different genetic information). Offspring that result from the union of gametes from male and female plants with dissimilar genotypes are known as hybrids. Plant breeders select genotypes (i.e., male and female parents) that complement each other to combine the positive (or desirable) traits from each parent in the hybrid offspring.
Genetic information is transferred from generation to generation through seed, which typically consists of an embryo, an endosperm, and a seed coat. The endosperm includes the beginnings of a radicle, a hypocotyl, and one or two cotyledons (seed leaves), which support the formation of roots, stems, and true leaves, respectively. Monocot plant species have a single cotyledon (i.e., mono = one) and dicot plant species have two cotyledons (di = two). The seed coat serves as a protective coat around the seed.
Plant Reproductive Systems
Autogamous Mating System
Crops are capable of both self- and cross-pollination. These crops are classified as either autogamous (self-pollinated) or allogamous (cross-pollinated) depending on the relative frequency of self- or cross-pollination that is observed in the species.
Self-fertilization occurs if male and female gametes derived from the same plant unite. Self-fertilization also refers to the union of gametes from the same genotype. One trait, i.e., plant characteristic, that virtually ensures self-pollination is cleistogamy, where pollen shed occurs before the flower opens (i.e., anthesis). Cleistogamy promotes self-pollination and severely limits cross-pollination. Cleistogamy is observed in some legumes (e.g., groundnut, peas, some beans, soybean). In some cereals (e.g., rice, wheat, and barley) the majority of self-pollination occurs before flowers open, but some cross-pollination can occur after the flowers open, even if only partially. This allows for some cross-pollination compared to relatively little or no cross-pollination in cleistogamous species. Most self-fertilizing species undergo a small amount of outcrossing: for example in soybean natural outcrossing of 0.03% to 2% or higher has been observed in some conditions (Caviness, 1966; Ray et al., 2003). Thus, it is critical to understand the mating system of the crops you are working with and how it is affected by different environmental conditions.
Examples of important crops with an autogamous mating system include: sorghum, millet, rice, wheat, barley, groundnuts, cocoa, and major pulse crops like cowpeas, and dry beans.
Allogamous Mating System
Fertilization in cross-pollinated plants occurs via the union of male and female gametes from different plants, which are often different genotypes.
A crop is classified as allogamous when it has a higher percentage of pollination and fertilization with different individuals than with itself.
There are several traits/plant characteristics that promote cross-pollination:
- Male sterility: Male sterility is caused by the formation of non-functional pollen grains, which prevents self-pollination and promotes cross-pollination. Two types of male sterility are: cytoplasmic male sterility (CMS), which is caused by mitochondrial genes interacting with nuclear genes; and genic male sterility (GMS), which is caused by nuclear genes alone. These phenomena can be exploited for hybrid seed production.
- Self-incompatibility: Self-incompatibility refers to the inability of viable pollen to fertilize flowers of the same or similar genotype. Self pollen is rejected on the surface of the stigma or in the style while foreign pollen is unaffected and can germinate, grow, and fertilize the egg cell. Self-incompatibility prevents self-pollination and enforces cross-pollination. More details on self-incompatibility is found in the Crop Genetics – Controlled Hybridization Module.
- Imperfect flowers: Imperfect flowers are missing either stamens or pistils (i.e., unisex flowers). Unisex flowers either occur on the same plant (i.e., monoecious) or different plants (i.e., dioecious).
Monoecious plants have separate male (i.e., staminate) and female (i.e., pistillate) flowers, although they occur on the same plant. In some crops, the male and female flowers are present in the same inflorescence such as in banana (Fig. 4). In some cases, they are on separate inflorescences, as in maize (Fig. 5).
Dioecious plants have separate staminate and pistillate flowers present on different plants. Dioecious plants are diclinous (i.e., having flowers of only one sex). Examples of dioecious crops include papaya, date palm, and spinach.
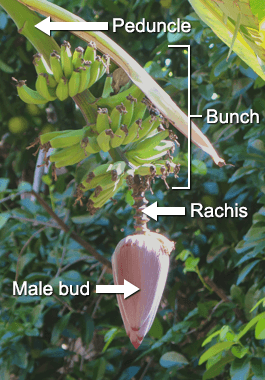
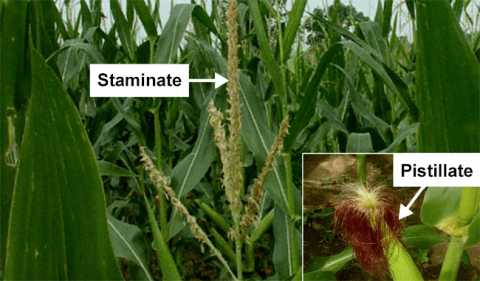
Protandry/Protogyny
- Protandry/Protogyny: Cross-pollination is often observed in crop species that show protogyny (i.e., the pistils/stigmas of a plant mature and become receptive before the anthers of that plant) and protandry (i.e., stamens/anthers of a plant develop and the pollen grains mature and are shed before the pistils/stigma of that plant mature and become receptive). Protogyny is typical in cassava (although this crop is not typically seed propagated) (Fig. 6), and it has also been used to make hybrids in pearl millet (Andrews et al., 1993). Sunflower and coconut are examples of crops that display protandry. Mechanical obstructions such as a membrane around the anthers in alfalfa (also called Lucerne) flowers may also limit normal dehiscence of pollen and limit self-pollination.
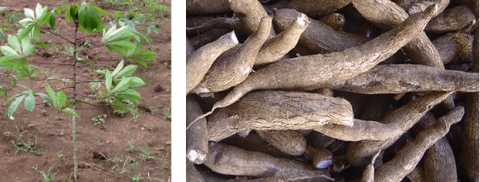
Understanding Reproductive Systems
Breeders must understand the reproductive system of the crop they are working on to make knowledgeable decisions about which breeding methods (i.e., crossing techniques, population maintenance, isolation distances, line and population development) are suitable and which type of cultivar (i.e., hybrid, pure-line, synthetic, clone) is appropriate. The modes of pollination and reproduction of some major crops are shown in Table 2. More information on the reproductive systems of crops is found in Allard (1960; pp. 40-41).
Mode of pollination and reproduction | Some examples of crop plants |
---|---|
A. Autogamous Species | |
1. Seed Propagated | Rice, wheat, finger, millet, barley, oats, common bean, cowpea, tomato, groundnut, soybean |
2. Vegetatively Propagated | Potato |
B. Allogamous Species | |
1. Seed Propagated | Maize, rye, pearl millet, sunflower, oil palm |
2. Vegetatively Propagated | Sugarcane, coffee, cocoa, banana, cassava, yam, rubber |
C. Autogamous species with cross pollination | Sorghum, cotton, pigeon pea |
Breeding Populations and Cultivar Types
Populations
Genetically speaking, a population is a group of individuals that share a common gene pool. If all individuals within the population have the same genotype the population is homogeneous; if the individuals have different genotypes the population is heterogeneous. For example, gene A has alleles A1 and A2 (assuming a diploid). If a population is homogenous then all individuals are the same; all are A1A1 (homozygous), or all are A1A2 (heterozygous), or all are A2A2 (homozygous). If a population is heterogeneous then some individuals have different genotypes; a combination of A1A1, A1A2, and/or A2A2.
The genotype of a population and individuals within a population varies depending on the reproductive system of a species. A natural population of a cross-pollinated species consists of a heterogeneous mixture of individuals some or most of which will be heterozygous (A1A2) for individual loci. A natural population of a self-pollinated species will usually also consist of a heterogeneous mixture of individuals, but each individual will be mostly homozygous (A1A1and/or A2A2) at individual loci. Populations of an asexually reproducing species may be homogeneous or heterogeneous and individuals will likely be heterozygous (A1A2) at many loci.
Cultivars
Some examples of populations are (1) a commercial maize hybrid cultivar (allogamous) which is homogeneous (single cross hybrid) and heterozygous, (2) a commercial soybean pure line cultivar (autogamous) which is homogeneous and homozygous, (3) a commercial maize synthetic cultivar which is heterogeneous and heterozygous, and (4) a commercial potato cultivar (clonal), which is homogeneous and heterozygous.
Clonal, synthetic, and hybrid cultivars are heterozygous. Pure-line cultivars are homozygous. Self-pollination is used to achieve homozygosity in an autogamous species. In allogamous species self-pollination is used to develop inbred lines that are used as parents to create hybrids
Clonal Cultivars
Clonal Cultivars: Most commercial crops are propagated through seed. However, a significant number of agriculturally important species are propagated by using plant parts other than seed which include: stem cuttings, suckers, tubers, and stolons (Fig. 7). As the term ‘clone’ implies, offspring are identical to the mother parent clone plant and are therefore homogenous in the absence of pollination and mutation. Clonal cultivars, although homogenous, are typically heterozygous, therefore ‘hybrid vigor’ is fixed and maintained, unlike a maize hybrid, which is propagated through seed, and loses hybrid vigor with each generation of selfing or sib-mating.
The steps to create/develop a clonal cultivar are:
- develop a genetically variable base/source population;
- evaluate and select superior clones from the population; and
- multiply the new cultivar for commercial use.
Examples of clonal cultivars include: cassava, sweet potato, potato, cacao, and yam. The cultivars of these crops are homogeneous and heterozygous

Synthetic Cultivars
Synthetic cultivars: Synthetic cultivars are produced by intermating a population of purposefully selected inbred lines, clones, hybrids, strains, or other populations of cross-pollinated plants. Synthetic cultivars are highly heterozygous and heterogeneous. Inbreeding depression is severe and plants that develop from self-pollinated seed lack the vigor of those obtained by cross-pollination. In a heterogeneous population, each plant is genetically different from another.
The components (clones, inbred lines, etc.) of a synthetic cultivar are maintained in their original form so that the cultivar can be reconstituted as needed. A synthetic cultivar is different from an open-pollinated variety because the components are maintained in their original form while with an open-pollinated variety the components are not maintained. Clonally propagated plants or inbred lines with desirable characteristics (traits) are selected and then isolated and allowed to cross-pollinate, randomly or in a structured format in a polycross nursery (Fig. 8). Seed is harvested from the clones or inbred lines and planted in progeny rows for evaluation. The best clones or inbred lines are then selected both for superior plant traits and on the performance of their progeny rows, which measures their general combining ability with the rest of the population. These selected parents are then replanted and permitted to cross pollinate in isolation. Open-pollinated seed harvested from these parental clones or inbred lines (after one or more cycles of intermating) is then sold as a synthetic cultivar.
Cultivar Development Example
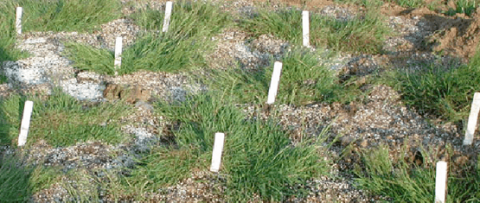
An example of the development of maize synthetics is the development of the maize population HIS1 (Brewbaker, 2009).
The development of synthetic cultivars of maize and other crops differs from that described for turfgrass and forage species because of several important biological differences.
- It is possible to inbreed maize because inbreeding depression is much less than in turfgrass and forage species.
- There is no self-incompatibility in maize and some self-pollination can occur, even though it is a monoecious species that is wind pollinated.
- It is an annual crop.
Pure-Line Cultivars
Pure-line cultivars: Pure-line cultivars are developed for self-pollinated species. Self-pollination leads to homozygosity and homogeneity.
Improved self-pollinated cultivars are obtained through three basic approaches.
- Introductions: a breeder assembles cultivated varieties currently grown in other regions (domestically or internationally) and identifies lines that exhibit desirable characteristics and are adapted to the new target area. A breeder must be aware of licensing and material transfer agreement (MTA) issues (see next module) before acquiring lines from different sources. Introductions are typically received as pure-lines, but they may contain mixtures of different genotypes or off-types and may require that the breeder rogues these plants out to obtain clean and uniform seed that can be released commercially. [Note: problem of mixture or off-types is common for self-pollinated, cross-pollinated or clonal crops; MTA are required for most if not all crops (self- and cross-pollinating and clonal) when the seed or explant is sent or acquired by breeders].
- Selection: a breeder assembles landraces, identifies the best genotypes, and releases one or more for commercial production. This approach has applicability for orphan crops but has not been widely used for major world crops.
- Hybridization: a breeder makes hybridizations between genotypes with desirable characteristics, evaluates, and selects superior genotypes for commercial production. This is the most common approach for developing new cultivars.
Cultivar Examples
Examples: beans, cowpea, rice, finger millet, tobacco, and wheat. Cultivars are homogeneous and homozygous.
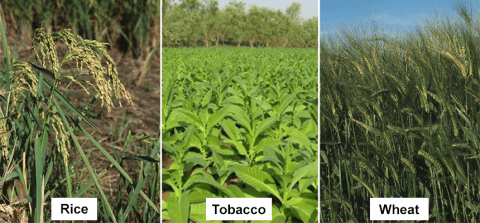
Hybrid Cultivars
Hybrid cultivars: Hybrid cultivars are produced by crossing inbred lines, typically two inbred lines in the case of a two-way/single-cross hybrid. Inbred lines are chosen for their combining ability to achieve maximum expression of hybrid vigor (i.e., the F1 trait mean is higher than the mid-parent mean and higher than the trait values for the individual inbred parents). Hybrid vigor (i.e., the visible measure of heterosis) is more important in allogamous crop species as the expression is typically lower in autogamous species. Pollen control via the use of mechanical tools, chemicals, or genetic male sterility is necessary to create hybrid seed.
Heterosis (or hybrid Vigor) is defined as the difference between the hybrid and the mean of the two parents (Falconer and Mackay, 1996). This definition is also described as midparent heterosis. High-parent heterosis is the superiority of a hybrid over the better parent (Bernardo, 2014).
Examples of hybrid cultivars include: commercial single-cross maize hybrids, commercial three-way cross maize hybrids, and sunflower hybrids. Hybrid cultivars are usually utilized for allogamous species but some hybrids are produced for some autogamous species (e.g., sorghum, tomato). Single-cross hybrid cultivars are homogeneous and heterozygous (Fig 10). Three-way hybrids are both heterogeneous and heterozygous.
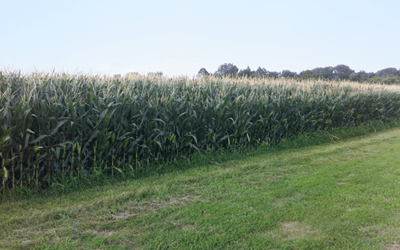
Seed Law
Definition of a Cultivar According to UPOV
According to seed law a cultivar or cultivated variety is distinct, uniform, and stable.
- Distinct: a cultivar is distinct if it can be differentiated by one or more identifiable characteristics from all other cultivars currently available or previously developed. Distinctiveness can involve morphological, physiological, molecular, or other characteristics.
- Uniform: a cultivar is uniform if no variation among individuals exists for the distinguishing characteristics that make it distinct from other cultivars.
- Stable: a cultivar is stable if plants remain the same from generation to generation. If there are multiple generations in a cycle of propagation the plant characteristics should be the same at the end of each such cycle.
These concepts come out of ‘The International Union for the Protection of New Varieties of Plants’ (UPOV), which is an intergovernmental organization based in Geneva, Switzerland. UPOV was established in 1961 by the International Convention for the Protection of New Varieties of Plants (the “UPOV Convention”).
Below is an excerpt from The International Union for the Protection of New Varieties of Plants (UPOV):
“The mission of UPOV is to provide and promote an effective system of plant variety protection, with the aim of encouraging the development of new varieties of plants, for the benefit of society.”
International Union for the Protection of New Varieties of Plants (UPOV) Criterion
Distinctness (Article 7)
Criterion: A variety is deemed to be distinct if it is clearly distinguishable from any other variety whose existence is a matter of common knowledge at the time of filing of the application.
Uniformity (Article 8)
Criterion: A variety is deemed to be uniform if, subject to the variation that may be expected from the particular features of its propagation, it is sufficiently uniform in its relevant characteristics. The uniformity requirement within the Convention has been established to ensure that the variety can be defined as far as is necessary for the purpose of protection. Thus, the criterion for uniformity does not seek absolute uniformity and takes into account the nature of the variety itself. Furthermore, it relates only to the relevant characteristics for the protection of the variety.
Stability (Article 9)
Criterion: A variety is deemed to be stable if its relevant characteristics remain unchanged after repeated propagation or, in the case of a particular cycle of propagation, at the end of each such cycle. As with the uniformity requirement, the criterion for stability has been established to ensure that the identity of the variety, as the subject matter of protection, is kept throughout the period of protection. Thus, the criterion for stability relates only to the relevant characteristics of a variety.”
Read the UPOV Overview online and go through the table of contents (under FAQs) to familiarize yourself with plant variety protection and plant breeder’s rights:
- What is UPOV?
- What is a plant variety?
- Why do farmers and growers need new plant varieties?
- How are new plant varieties of benefit to society?
- What is Plant Variety Protection?
- Who can protect a plant variety?
- What are the Exceptions to the Breeder’s Right?
- What are the conditions for obtaining protection?
- What information is there on the impact of PVP?
Visit the main UPOV website for more details on UPOV.
Setting Breeding Objectives
Plant breeding objectives will depend on geographical adaptation, prevalent biotic and abiotic factors that influence production, uses of a cultivar, crop reproductive system (for example, pureline or hybrid), and factors that are important to farmers, and end-users. It is fundamental that genetic variation exists for the trait that you are attempting to improve and that it is transmissible. Plant breeding programs need to be adequately set up for screening breeding material for the traits that are being improved based on the objectives. Applied learning activity #1 will be used to better understand the considerations involved in setting plant breeding objectives.
References
Andrews, D.J., B. Kiula, and J.F. Rajewski. 1993. The use of protogyny to make hybrids in pearl millet. p. 122-126. In: J. Janick and J.E. Simon (eds.), New crops. Wiley, New York.
Allard, R.W. 1960. Principles of plant breeding. John Wiley and Sons, New York.
Bernardo, R. 2014. Essentials of Plant Breeding. Stemma Press, Minnesota.
Brewbaker, J.L. 2009. Registration of nine maize populations resistant to tropical diseases. Journal of Plant Registrations 3:10–13.
Caviness, C.E. 1966. Estimates of natural cross pollination in Jackson soybeans in Arkansas. Crop Sci. 34:376–378.
DePauw, R.M., R.E. Knox, F.R. Clarke, H. Wang, M.R., Fernandez, J.M. Clarke, and T.N. McCaig. 2007. Shifting undesirable correlations. Euphytica 157:409–415.
Falconer, D. S., and T. F. C. Mackay. 1996. Introduction to quantitative genetics. 4th ed. Longman, Essex, England.
FAO 2014. Major African crops. Statistics Division (FAOSTAT, 2014).
Ngopya, F. 2003. Importance of root crops in Africa. In Proceedings of the Expert Consultation on Root Crop Statistics – Volume II: Invited Papers. Harare, Zimbabwe, 3-6 December 2002. Food and Agriculture Organization of the United Nations, FAO, Rome.
Ray, J.D., T.C. Kilen, C.A. Abel, and R.L. Paris. 2003. Soybean natural cross-pollination rates under field conditions. Environ. Biosafety Res. 2:133–138.