Chapter 6: Breeding Methods
Asheesh Singh; Arti Singh; Anthony A. Mahama; and Walter Suza
The results of breeding and selection may be new varieties or clones that are superior to currently used standard commercially grown genotypes (checks) according to some criterion or criteria, or populations that are superior to previous ones. Several breeding strategies exist and though some methods are generally commonly accepted, different methods are applied in different crops as they are more efficient and effective based on the type of mating of different crops, resources and objectives. In other words different breeding strategies are deployed and used to maximize superiority per unit cost and time. Also depending upon the goals of the breeding program, different strategies may be used simultaneously or at different stages of the program.
- Identify and describe different plant breeding methods relevant to crops grown in Africa
- Mention and describe innovation used to enhance backcross breeding method
- Explain innovation used to enhance recurrent selection method
Methods Used in Self-Pollinated Crops
In self-pollinated crops, the following breeding methods are commonly used to develop pure-line cultivars:
- Bulk method
- Pedigree methods
- Single Seed Descent
- Doubled Haploid
Example of self-pollinated crops in which these methods are used include: common bean, soybean, cowpea, groundnut, rice, wheat, barley, millet, and sorghum.
In specific situations, for example, when a breeding program is converting pure-lines to contain a specific gene or 2-3 genes (of qualitative inheritance), the backcross breeding method is used.
The doubled haploid method is not used in legume crops as these species have so far been recalcitrant to tissue culture and haploid induction and rescue.
Methods Used in Cross-Pollinated Crops
In cross-pollinated crops, the following breeding methods are used to develop cultivars:
- Recurrent selection (for example, maize)
- Development of hybrids: a 2-step process where first inbred lines are developed and assessed for their specific combining ability, followed by crossing of the inbred lines (generally, 2 inbred lines, but can be 3 or 4) to produce hybrid, as for example, maize, rice, sorghum, cotton.
Few self-pollinated species (such as rice, sorghum, and cotton) have some level of outcrossing and expression of heterosis, which is exploited to develop hybrid cultivars.
Recurrent selection methods are used to develop open-pollinated varieties or synthetics.
Methods Used in Clonal Crops
The crop species that can be clonally propagated present unique advantages:
- Heterosis can be fixed in F1 and in subsequent crop production cycles, and its clones can be propagated to preserve the high yield advantage.
- Farmers can harvest the crop and use the vegetative plant part to grow the next crop. For example, potatoes, sugarcane, cassava.
In breeding clonal cultivars, hybridization is made between two clones and a large F1 population (remember that parental clones are heterogeneous and heterozygous) is screened as each F1 is unique and different from other F1s. This process is repeated over different crop cycles to identify the superior clone for release as a new cultivar.
Breeding Methods Used in Major Crops
Pedigree Method
The pedigree method of breeding is used in development of both self-pollinated (to develop pure-lines) and cross-pollinated crops (to develop inbreds). It is one of the most commonly used breeding methods. Selection of highly heritable traits is practiced in early generations on individual plants. Yield testing is generally done once homozygous lines are developed (Fig. 1). However, in an early generation testing procedure or a modified pedigree method, yield testing is done in early generations while within-family selection is still ongoing.
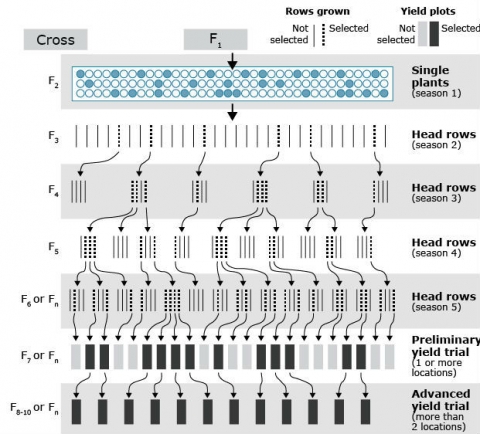
Explanation of steps in Fig. 1
-
- Select in F2 and later generations.
- Selected F3 plants (or seed from inflorescence of selected plants) grown in next season (in winter nursery if available).
- Selected F3 rows (or selected plants within rows) grown as F4 in rows (or yield plot).
- Selected F4 plants (or seed from inflorescence of selected plants) grown in next season (in winter nursery if available) as F5.
- Repeat this process until selection is effective (remember, additive genetic variance among lines increases but decreases within lines as selfing is used).
- Bulk harvest the last generation when a row is grown (and appears homogenous), F6 or Fn and plant in the next season as a yield plot.
- Grow through successive seasons of yield testing to select the genotypes that are superior to checks.
- Pedigree information is kept to maintain family information, which allows selecting more plants from families that are superior performing or to advance families for yield testing if those families are superior.
Additional notes
- Number of plants/row and population sizes vary between programs and some estimates can be obtained from text books or plant registration documents. These numbers will depend on the objective of the cross, number of crosses made per year, available resources (technical, infrastructure).
- Selection for other specific traits is simultaneously happening (on harvested seed, or specific nurseries).
- Single plants or inflorescence per plant are selected at each generation, but in some visibly inferior rows, breeder may not make any within rows selection (i.e., practice among row selection).
- Selection can be practiced in winter nursery if genetic correlation is high among home location and off-season location (i.e. winter/dry season nursery locations).
- A breeder may combine two or more methods of breeding and these methods will then be called modified pedigree (or modified bulk, or modified single seed descent etc.).
Bulk Method
Bulk method allows natural selection to act and remove undesirable genotypes from the population (i.e., per cross) (Fig. 2). The choice of growing environment will dictate what kinds of traits will be selected for or against, therefore care needs to be exercised to use environments that are suitable for realizing the objectives of the program.
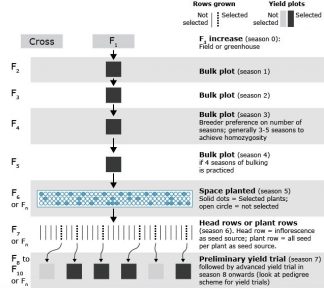
Explanation of steps in Fig. 2
- Generations are advanced to homozygosity through bulks.
- It is a low cost, less technical method of breeding.
- Natural selection is used to remove undesirable plants.
- Artificial selection environment can be used to select for a trait of interest. Bulks can be grown in a disease or another stress nursery to select for that trait. Markers can also be utilized to select for desirable traits to constitute the bulks. These variations will make the scheme as a modified bulk method.
- Early generation testing of bulk may be done for yield testing and to make a decision on retention of populations based on ranking among populations.
Additional notes
- In modified bulk method, single plants or inflorescence per plant are selected at each generation; while in bulk method, plants from the entire population are harvested and seeded (all or sub-sample of seed) in next generation.
- Lighter shade yield plot = grown, tested, not selected; darker shade yield plot = grown, tested, selected and advanced to next generation testing.
Single-Seed Descent Method
Single Seed Descent (SSD) was developed as a breeding method to rapidly advance lines to homozygosity so that selection can be practiced on homozygous lines (Fig. 3). The original intent of this method was to maintain a large population size to mimic the genetic variation in F2 generation for effective selection. However, this method is now used to reduce the time to develop cultivars. (Sleper and Poehlman, 2006).
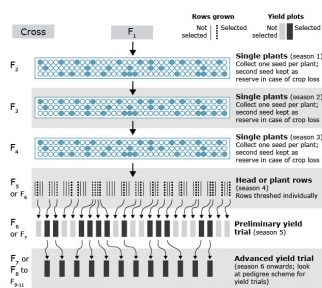
Explanation of steps in Fig. 3
- Generations are advanced to homozygosity rapidly. In case of small grain crops (such as wheat, barley, oats), three seasons can be completed in artificial growing conditions (greenhouse etc.), and limited space is needed to keep a population size of 250-300 seed per cross.
- If true single seed descent is practiced (where one seed per plant is grown in successive generations, population size is reduced in each cycle due to losses due to no germination and emergence. As an alternative modified, single seed descent can be used where 2-3 seed per plant are planted in hill plots in each cycle, and 2-3 seed from each hill are collected from an inflorescence.
- SSD plots can be grown in a disease or another stress nursery to select for that trait.
- It is a cheaper, less technical method of breeding. Rapid inbreeding and homozygosity is achieved.
- No need for record keeping of individual plants while advancing through SSD.
- Open circle = single plants (or hills in modified SSD) per population.
Doubled Haploid Method
Doubled haploids (DH) are created by generating haploid plants from microspores (androgenesis) or unfertilized eggs or ovules (gynogenesis). Haploid plants are then subjected to a chemical treatment (with colchicine) to double their chromosome number to produce homozygous diploid plants.
Doubled haploids are generated from heterozygous plants, typically F1 plants derived from crossing of two pure-lines or inbred lines. DH can also be developed from selected F2 individuals from a cross. This method is used in development of both self-pollinated (to develop pure-lines) and cross pollinated crops (to develop inbreds). Process is shown in Fig. 4.
- Generations are advanced to homozygosity in single generation. DH genotypes are true homozygous.
- Specialized lab is needed to create doubled haploids. Can be generated through a service provider.
- Population size is an important consideration because only one generation of meiosis occurs (at F1).
- This method is suitable for marker assisted breeding to select for traits that are fixed.
- Can develop cultivars most quickly. If sufficient seed is available, can go to advanced yield trial in season 3.
- It is becoming a preferred method of inbred line development in maize.
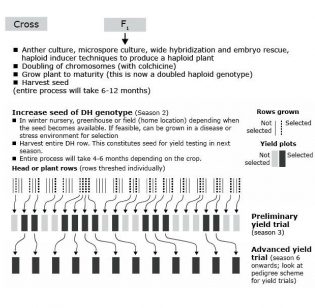
Backcross Breeding Method
The backcross breeding method is used if the objective is to introgress a gene into an elite cultivar or breeding line. Examples are disease resistance gene(s) and herbicide tolerance gene(s) (Fig. 5). By crossing to the recurrent (adapted) parent, the newly developed cultivar will contain the majority of the recurrent parent genome and only the gene of interest from the donor parent.
If the gene to transfer is recessive (rr), progeny of crossing with RR recurrent parent will segregate as RR and Rr, and therefore progenies are selfed for one generation to determine the Rr type versus RR types (RR are discarded) before making the next backcross. With the application of molecular markers, this extra step has become redundant and F1 plants can be grown, DNA extracted from young plant tissue to determine Rr and RR types. RR types can be removed and crosses can be made with Rr types.
For a backcross breeding program, if the gene to be moved comes from an unadapted or related species, the breeder has to be aware of inadvertently bringing in undesirable genes linked to the desired target gene (termed linkage drag). Larger population sizes will need to be grown to identify recombinants. Innovations, e.g. marker assisted backcrossing, marker assisted recurrent selection, and genomic selection, exist that reduce the need for large population sizes.
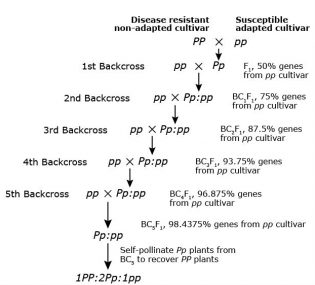
Innovations in Backcross Breeding
Marker-Assisted Recurrent Selection
Reliability for Selection
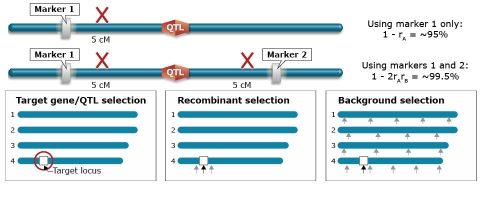
Steps of Marker-Assisted Recurrent Selection
- One generation of phenotypic selection in the target environment is conducted,
- Markers with significant effects are used to predict the performance of individual plants, and
- Several generations of marker-only selection are performed in a year-round nursery or greenhouse
Comparison between Conventional and Marker-Assisted Backcrossing
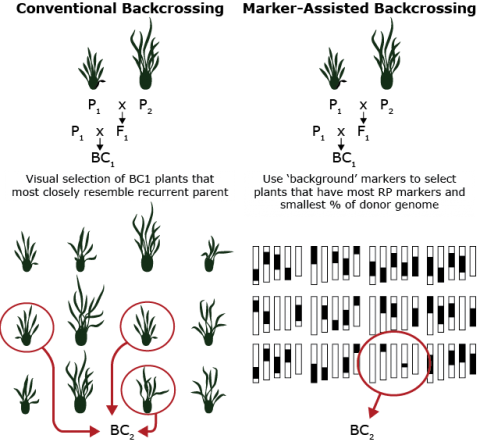
Early Generation Testing
Early Generation Testing (EGT) describes the procedure for selecting superior lines or families before they are homozygous. It also refers to a specific use where a genetic worth of a population is determined by analyzing yield data from a segregating (early generation) plot and removing entire populations. EGT is used in self- and cross-pollinated species.
In the pedigree breeding method we looked at individual plant selection for highly heritable traits in early generations. With high heritability, individual plant selection is still effective, for example traits such as plant height, disease resistance, and morphological traits. Several breeding programs, however, follow a modified method (such as modified pedigree method), in which yield testing is started in an early generation (for example, F3 or F4) to make selections. The early generation lines are grown on yield plots (2 or 4 row plots), therefore, more resources are required to handle EGT. Nonetheless, EGT allows elimination of materials (lines) that are inferior due to use of replication and multi-environment testing. Also, selection for lower heritability can be practiced to discard inferior lines.
Other breeders may choose to perform a yield test on populations derived from early generation bulks to identify superior bulks (inferior bulk populations are removed completely from further generation advancement). Thus, EGT testing in this scenario can be done for one or 2 generations followed by selection of superior plants, and then starting yield testing of these lines.
Cytoplasmic Male Sterility Systems
Plant breeders working with cytoplasmic male sterility (CMS) systems will aim to develop new ‘B-lines’ and ‘R-lines’. In crops where CMS system is used to produce hybrids, different ‘R’ restorer genes are identified and breeders will improve ‘R-lines’ that will be used as males in creation of hybrids. ‘B-lines’ and ‘R-lines’ are developed using the self-pollinated breeding methods we learned about earlier in this module (pedigree, bulk, SSD, DH etc., or a modified method that combines more than one method in the development of breeding line of cultivar).
An outline of a CMS system is shown in Fig. 8. Note that the ‘R’ and ‘r’ genes are in the nucleus and the ‘S’ and ‘F’ genes are in the cytoplasm.
A breeder who develops ‘B-lines’ will use the backcross method to develop ‘A-lines’ using available CM sterility genes. A hybrid cultivar is produced by crossing of ‘A-lines’ with ‘R-lines’. The A/B and R gene pools are considered separate gene pools (reproductive gene pools) similar to heterotic gene pools we learned about in maize systems.
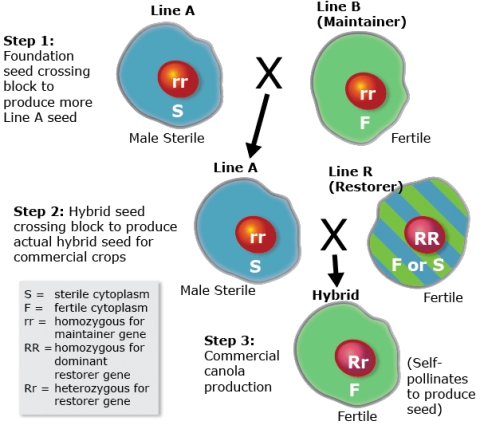
Hybrid Cultivars
In the chapter on Steps in Cultivar Development, we looked at the development of maize hybrids using two-way crosses. Crosses are made within a heterotic group to develop superior inbred lines in the heterotic group. These inbred lines are crossed to testers from other heterotic groups to decide on the best specific combing ability. This process is repeated for all heterotic groups that the breeding institution or company works with internally.
For evaluation, superior inbred lines from dissimilar heterotic groups are crossed to produce hybrids. Several 100 or 1000’s of hybrids are evaluated each year to finally pick the most superior hybrid(s) for commercial release based on their performance and target area of adaptation (maturity, stress, environment etc.).
Hybrid seed is produced by growing inbred female rows (say 6 to 8) from one heterotic group and inbred male rows (1 or 2) from a dissimilar heterotic group interspersed among the sets of female rows, and de-tasseling the female rows (that is, removing male inflorescences from female plant rows) before pollen shed. Manual or mechanical tools are used to de-tassel (prior to pollen being ready or shed to avoid any selfing of plants of the inbred female line). Cobs from female rows are harvested and these constitute the hybrid seed. In some programs, but routinely done in private seed industries, the male rows are usually destroyed when pollination is completed to avoid contamination from cobs from inbred male plants if allowed to grow and produce cobs.
Recurrent Selection
In recurrent breeding and selection, parents of a crop species are crossed to develop populations using various mating designs described in the chapter on “Refresher on Population and Quantitative Genetics.” Based on one or more selection criteria, and using within family and among family selection strategies, individuals are selected and inter-mated to produce the next generation. This procedure of selection can continue for an indefinite amount of time, hence the term “recurrent”. Recurrent selection method is employed in order to achieve the following:
- The goal of recurrent selection is to improve the mean performance of a population of plants and to maintain the genetic variability present in the population.
- The underlying principle of recurrent selection is to increase the frequency of desirable genes that the breeder is attempting to improve.
- Recurrent selection is used to improve populations in cross pollinated species. Open pollinated varieties are one type of cultivar developed using recurrent selection.
Comparison: Mass Selection versus Phenotypic Recurrent Selection
- Mass selection: Female plants are selected after pollination with unselected and selected pollen source.
- Phenotypic recurrent selection: Male and female are both controlled. ONLY selected plants are intercrossed to obtain seed for the next cycle of selection. Expected genetic gain from selection of only the female parent is one-half compared to expected genetic gain when both parents are selected.
Note that the terms mass selection and phenotypic recurrent selection are sometimes used interchangeably and one would have to look at the breeding scheme for details in order to determine which method is being referred to.
Comparison: Genotypic versus Phenotypic Recurrent Selection
The difference between genotypic and phenotypic recurrent selection is that Genotypic Recurrent Selection is selection based on progeny performance (combining ability), while Phenotypic Recurrent Selection is selection based on the phenotype of the individual.
Examples
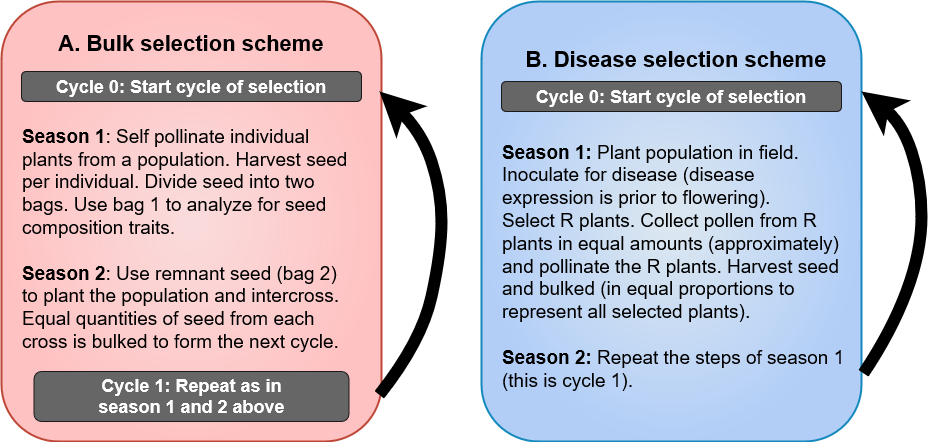
Phenotypic Recurrent Selection Issues
There are several problems with selecting individual plants in the field:
- Micro-environment variability does not permit assessing breeding value.
- Competition effect due to uneven planting.
Solutions to these problems include:
- Gridding designs (selecting plants within a grid)
- Not selecting plants that have missing neighbors
The generalized recurrent selection method consists of the following steps:
- development of a base population (for selection).
- evaluation of individuals from the population
- selection of superior individuals from the population
- intercrossing the selected individuals to form a new population.
Development of Base Population
A base population can be an existing population (for example a maize synthetic) which may not have been previously selected for your trait of interest.
More commonly, a base population will come from outstanding families from a recurrent selection program. It may also be created with elite inbred lines. Smaller number of inbred lines will ensure use of elite material that are similar morphologically, but inbreeding depression will be greater.
Superior inbred lines are identified based on their performance in multi-location tests and superior general combining ability (specific combining ability is not as important in the performance of OPV; it is most important if one is developing a hybrid cultivar).
These superior inbred lines are crossed using an appropriate mating design from among available designs (for example, diallel design).
Evaluation of Individuals
Individuals are evaluated for selection when advancing generations following crossing, and the type of cross made play a key role in the phenotypic and genotypic schemes employed in selecting individuals (Table 1).
Phenotypic scheme | Genotypic scheme |
Evaluation is based on individual plants per se | Evaluation is based on the performance of the progeny of the individual |
Assessment is very variable unless species can be clonally propagated | Progeny performance strategy allows for replicated, multi-location testing. |
Not easy to control environmental variability | This provides a more accurate assessment of individual’s breeding value |
n/a | Three types of progenies can be evaluated: self, full sib, or half sib |
Progeny are produced by self-fertilizing the individuals that are evaluated for selection.
Full-sib families are created by crossing the individuals to be evaluated in pairwise combinations. Since in each pairwise cross both parents are common for that family, individuals of that family are full-sibs.
Half-sibs are formed by crossing the individuals to be evaluated to a common parent (which can be a population or an inbred line as a tester. Since all progeny have the tester as a common parent, they are half-sibs.
Population Improvement
As the name implies, the breeding populations creating from crossing parents, need to be improved in performance of the desired traits, in order to continue to make progress in breeding programs. Different methods are used for population improvement, and depending on the breeding program’s specific project goals, can described as intra population or interpopulation improvement. Table 2 shows some methods that are used.
Intrapopulation improvement | Interpopulation improvement |
Mass selection (with or without pollen control) | Reciprocal half sibs recurrent |
Half-sib family | Reciprocal full sib |
Full-sib family | Testcross |
Selfed family | n/a |
Recurrent Phenotypic Selection
Steps include:
- Plant a population (space planting individuals to facilitate note taking on individual plants).
- Evaluate for trait of interest and identify the best individuals (higher heritability such as flowering time or morphological traits are suitable for this method).
- Harvest seed of the best individuals and reconstitute seed to form the next cycle of recurrent selection.
- In this example, pollen control can be exerted if the trait can be evaluated prior to flowering. Undesirables can be removed before they contribute pollen to the rest of population; and this ability to control parental pollen helps improve the response to selection.
Recurrent Half-Sib Selection
- An intra-population improvement method: cross the individuals in a population to a common tester (population per se, or inbred tester), evaluate the half-sib progeny of each plant, select the best individuals, and intercross the selected individuals.
- The main step is evaluation of individuals through their half-sib progeny. There are numerous variations within and among crops based on what is used as a tester (population vs inbred), parental control, intercrossing.
- Where possible, it is desirable to control both parents. This can be achieved by evaluating in one season and recombining in another generation (in winter nursery or second season). This necessitates an extra season but genetic gain per year will be higher. While the half-sib are being evaluated, the remnant seed of the individual needs to be kept as reserve so that this seed can be used if the individual is selected based on the half-sib performance to intermate and create material for the next cycle of selection.
- In maize, obtaining selfed and half-sib seed from the same plant can be accomplished by self pollinating the single ear on the individual to be tested and using pollen from that individual to pollinate several individuals of the tester (bulk of population per se, or inbred line). The ears on the tester, bulked together from that individual as pollen source, represent the half-sib family to be tested for that individual.
- Recombining selfed progeny will require three seasons: (1) selfing and crossing to the tester, (2) evaluation, and (3) intercrossing selfed progeny.
Recurrent Half-Sib Examples
Female parent selected; population used as tester.
- Start with a random mating population
- Harvest ears of each plant (say, 200). Grow 200 half-sib progeny plots (with checks) at multiple locations (can be unreplicated or replicated). Traits of interest is yield (for example). At one location, grow in isolation as seed source for the next cycle. At this location, select plants within a half-sib row. At other locations, use for testing.
- At the location with isolation, grow the male rows (bulk seed of all half-sib families) adjacent to female half-sib rows. De-tassel the female rows.
- At the location where grown in isolation harvest ears from each selected plant by hand. Make selections to pick the best half-sib families. These ears will form the next cycle seed.
- Season 2, conduct random mating of selected plants.
- Repeat steps
- One can use an inbred line as tester instead of bulk seed of population used as male.
Female and male parent selected; population used as tester.
Cycle 0: (intermate population)
- Harvest ears from each plant (selection may be performed)
- Divide the seed of half sib plants into two: part 1 for next season field testing, part 2 for remnant to reconstitute selected half-sibs.
Season 1: Each half sib (using part 1 seed) is a separate entry in replicated or unreplicated trials with 2 or more locations, with checks.
- Select superior half-sib families based on performance. These selections will be used in crossing.
Season 2: remnant seed (part 2 of seed bag) of selected individuals is used for intercrossing to form next cycle.
Cycle 1: Seasons 3 and 4 – repeat as above.
- One can use an inbred line as tester instead of bulk seed of population used as male.
Recurrent Half-Sib (Testcross Progeny)
- Start with an intermated population
- Season 1: plants in an intermated population are selfed and pollen used for selfing and pollinating a tester.
- Season 2: testcross progeny are evaluated in replicated tests. Selections made to identify superior performing progenies.
- Season 3: selfed seed of selected families are used to form the next intermating cycle. Cycle is repeated as above.
Recurrent Full-Sib Process
The main steps are listed below.
End of first year:
- Season 1: Make paired crosses between individuals in the population.
- Season 2: Evaluate the full-sib families in the field and identify the best families.
- Season 3: Recombine (intercross) the best families using remnant seed from the first season.
Start of second year:
- Season 4: Begin the second cycle with paired crosses between individuals in the population.
An advantage is the completion of one cycle per year. A disadvantage is less recombination between cycles of selection.
Recurrent Full-Sib Example
Start with an intermated population. Make selections.
- Season 1: paired crosses are made between pairs of selected plants in a population. Seed is divided into two parts: Part 1 is for field testing, and Part 2 is to reconstitute next cycle.
- Season 2: Part 1 seed used to plant field tests. Evaluate full-sib in field tests (single or multiple locations, unreplicated or replicated, with checks). Select superior families based on performance.
- Season 3: Part 2 seed used to intercross selected families. Intermated seed is used to form the next cycle.
- Cycle 2: Seasons 4, 5, 6.
Recurrent Selection Among Selfed Families
- Season 1: S0 plants from the population are selfed to produce S0:1 lines.
- Season 2: Evaluate the selfed progenies in field (for trait of interest).
- Season 3: Use the remnant S1 seed from season 1 to intercross selected lines.
This completes cycle 1 and S0 plants are obtained. The cycle is repeated as described above in season 4-6 for cycle 2, and so on.
Variation can include more than one generation of selfing if more seed is required for evaluation.
Reciprocal Recurrent Selection
Reciprocal recurrent selection (RRS), as a breeding method for open-pollinated crops was first proposed by Comstock et al. 1949 to take advantage of both additive and dominance genetic effects. In brief, plants from one population are mated to plants of another population, and selection of individuals for the next cycle of selection is based on the performance of progeny in hybrid combination. For this breeding method, each cycle requires one generation for selection of individuals and a second generation for intermating of selected individuals to produce materials for the next generation. RRS is a procedure to improve both the general and specific combining ability of two populations simultaneously, and steps involved are as below:
- Plants are selected in each of two populations
- Plants of population#1 are selfed and outcrossed as the tester to the selected plants in population#2 to generate test cross progeny.
- Plants of population#2 are selfed and outcrossed as the tester to the selected plants in population#1.
- The resulting test cross progenies are evaluate in each season. Superior plants are identified based on their test cross performance. Selfed seed from these selected plants are used to intercross within each population to generate materials for the next generation.
- Cycle is repeated.
Maize Open Pollinated Varieties (OPV)
Development of a Maize OPV
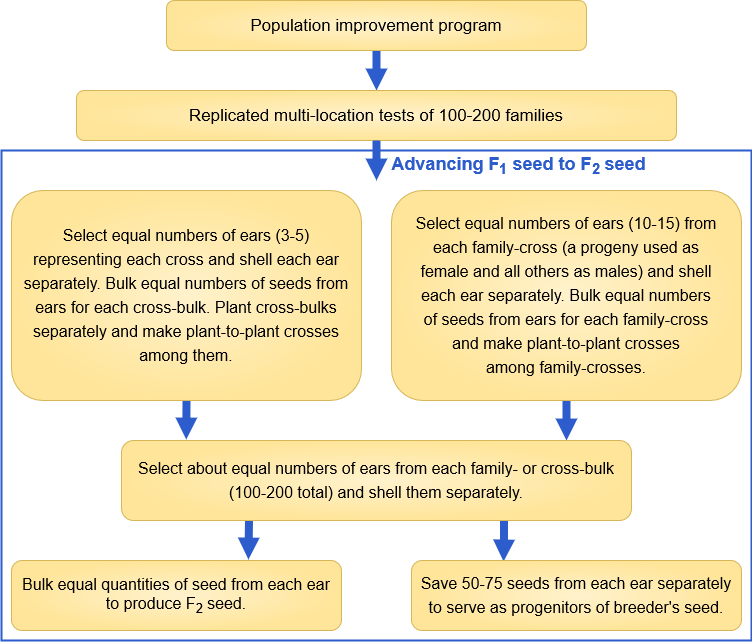
Maize OPV Cultivar Evaluation
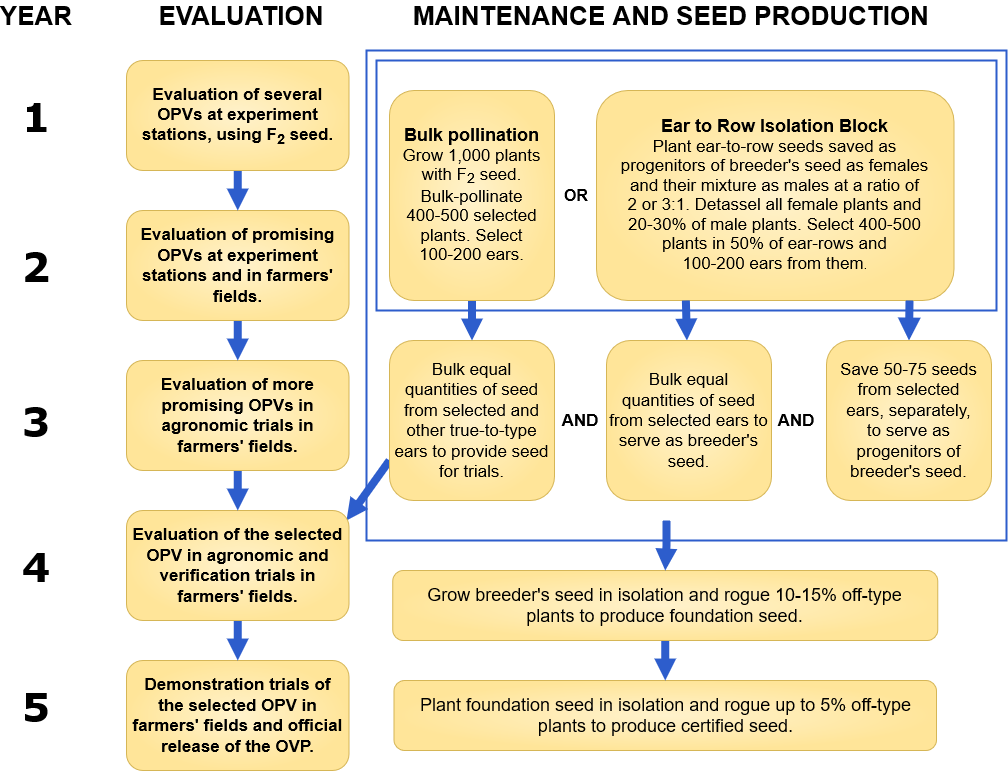
OPV Advantages and Disadvantages
ADVANTAGES | DISADVANTAGES |
Seed can be re-cycled (if grown in isolation or middle field harvested without a significant yield reduction due to inbreeding depression) | Yields lower than hybrids |
Can have much more broader adaptability compared to hybrids (that are developed for targeted areas) | Is not comparable to hybrids in areas where land is fertile and inputs are available to maximize yield |
May be less costly than hybrid | Plants are less uniform |
May require less inputs than hybrids | Seed needs to be harvested properly to use for next year, and even then there will be a yield reduction. |
OPV may be more accessible in areas where no hybrids are available or seed availability channels are poor | n/a |
Clonal Cultivar Methods
Since each clone breeds true (i.e., no gene segregation because no sexual recombination), breeding programs can evaluate a clone in several different tests simultaneously (field testing, disease nursery etc.). In clonal crop breeding, each cross produces unique and distinct F1 seed (true seed). True seed plants are transplanted into field testing and selection commences to identify which F1 of F1’s are suitable for cultivar release. Step-wise reduction process is used to discard undesirable F1 clones each testing season (remember, clones can be propagated for more extensive testing once smaller number of desirable clones are identified. Shown in Table 4 below is an example of sugarcane cultivar CP 03-1912 developed in Florida.
Year | Month | Stage and activity completed | Number of genotypes in stage | Locations |
---|---|---|---|---|
2000 | Dec | Cross made at USDA–ARS sugarcane field station | No data | Canal Point, FL |
2002 | May | Germinated true seed transplanted into field (seedlings) | 100,000 | Canal Point, FL |
2003 | Jan | Advanced from plant–cane seedlings to stage 1 | 15,000 | Canal Point, FL |
2003 | Sep | Assigned name CP 03-1912 in stage 1 | 15,000 | Canal Point, FL |
2003 | Nov | Advanced from plant cane stage 1 to stage 2 | 1,496 | Canal Point, FL |
2004 | Nov–Dec | Advanced from plant cane stage 2 to stage 3 | 135 | Four farms in Florida |
2006 | Nov–Dec | Advanced from plant cane stage 3 to stage 4 sand soils | 13 | Four farms in Florida |
2011 | Feb | Cultivar release | 1 | No data |
Approximately 10% culling rate was practiced in each season after 2003. As seasons advance, clones are grown in replicated yield trials at several locations and comparisons with standard checks is made to identify which clones to advance to the next stage of testing.
Synthetic Cultivar
Synthetic cultivars are formed by using clones of inbred lines in pre-determined proportions for released to farmers. Farmers can use a synthetic for several generations (as open-pollinated population) but once inbreeding depression causes yield reduction, farmers need to use seed from the breeding institution or company. Therefore synthetics are reconstituted regularly by the breeder. Maize is an example where synthetics have been developed. In crops with self-incompatibility, synthetics are the preferred types of cultivars as the method exploits heterosis for a few generations.
Clones or inbred lines used in the formation of synthetic are chosen on the basis of their general combining ability. Crossing is made to ensure random pollination allowing gametes of each component (clone of inbred line) to be equally represented.
References
Canola Council of Canada. 2014. Canola varieties.
The Maize Program. 1999. Development, Maintenance and Seed Multiplication of Open-Pollinated Maize Varieties – 2nd edition. Mexico, D>F.: CIMMYT.
Comstock, R. E, H.E. Robinson, and H.P. Harvey. 1949. A breeding procedure designed to make maximum use of both general and specific combining ability. J. Am. Soc. Agron. 41: 360-367.
Gilbert et al. 2011. Registration of ‘CP 03-1912’ sugarcane. JPR 5(3): 319-324.
International Rice Research Institute. 2006. Molecular breeding, Lesson 1: Marker assisted breeding for rice improvement.