Chapter 11: Rice Breeding
Arti Singh; Asheesh Singh; and Anthony A. Mahama
Rice is grown on all six continents in the world except Antarctica. The two cultivated rice species are Oryza glaberrima, commonly known as African rice, and Oryza sativa L., commonly known as Asian rice, which has two major subspecies (japonica and indica). Cultivated rice is a diploid species (2n=2x=24) with basic chromosome number of 12. Twenty-one different wild varieties exist. Rice is one of the most important food crops in the world and is the staple food in numerous countries, particularly in Asia.
- Become familiar with the rice crop
- Demonstrate knowledge about the crop’s biology and classification system
- Know the origin and domestication of the crop
- Outline the classification of the different production systems
- List breeding institutions working on the crop
- Discuss the breeding methods used to develop pureline and hybrid rice cultivars
Origin
Rice has three distinct cultivated species and 21 different wild varieties. Information on the origin of glaberrima and the two oryza subspecies is as below:
- Oryza glabberima – domesticated in West Africa between about 1500 and 800 BC or about 2,000-3,000 years ago (Linares, 2002).
- Oryza sativa japonica – domesticated in central China about 7000 BC or about 8,200-13,500 years ago (Molina et al 2011; Huang et. al., 2012; Harris, D. R., 1996; Vaughan et al, 2008)
- Oryza sativa indica – domesticated in the Indian subcontinent about 2500 BC (Londo et al, 2006)
Major Categories
Rice is grouped into four major categories worldwide:
- indica is the long-grain type and is non sticky when cooked
- japonica is the short-grain type which becomes sticky when cooked
- aromatic is the medium to long-grain type which when cooked has nut-like aroma and taste.
- glutinous is the type that is especially sticky and glue-like when cooked
Domestication and Diversity
Wild Ancestor of Rice
The wild ancestor of cultivated rice (Oryza rufipogon) existed over a broad range of geographic regions across Asia (Fig. 1). Domestication of O. rufipogon in response to human selection resulted in complete transformation of morphological and physiological traits of the plant. Consequently, cultivated rice (O. sativa) displays reduced dormancy, grain shattering and outcrossing, and reduced loss of pigmentation in the hull and seed coat.
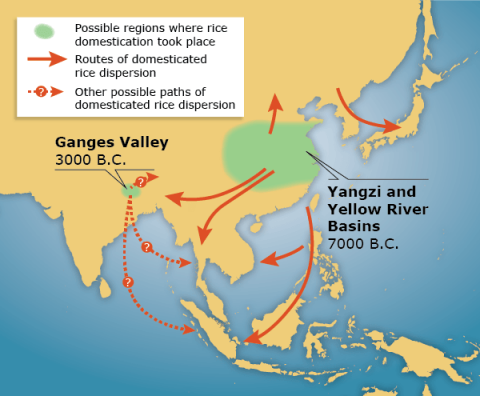
Transformation to Cultivated Rice
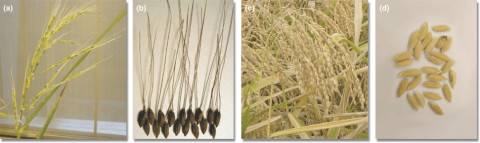
In addition, there is a better synchronization of tiller development and panicle formation in modern rice cultivars along with an increased number of secondary panicle branches (Fig. 2), higher grain yield and weight, and improved photoperiodic response.
NERICA
NERICA stands for “New Rice for Africa” developed using interspecific hybridization of O. glaberrima (African rice) and O. sativa (Indian rice) at the Africa Rice Center (WARDA) (Fig. 3). NERICA was developed for the purpose of raising the yield of African rice cultivars.
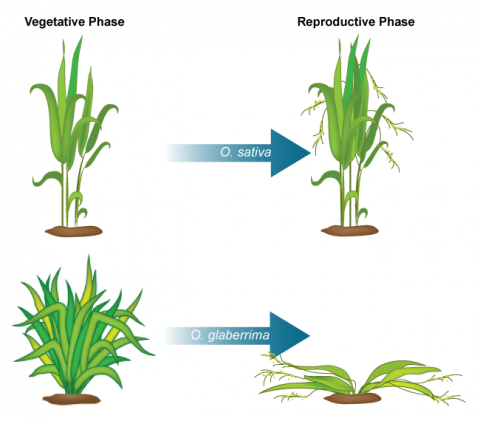
Since inter-specific crosses do not result in viable seed, embryo-rescue technique was used for the production of NERICA rice. The resulting hybrid rice cultivar has a higher yield due to increased grain size, better growth and also resistance to biotic (diseases and pest) and abiotic (drought) stresses. Dr. Monty Jones won the 2004 World Food Prize for creating a rice cultivar specifically bred for the ecological and agricultural conditions in Africa. The new rice cultivar was suitable to African drylands including and is grown in Guinea, Nigeria, Côte d’Ivoire, and Uganda.
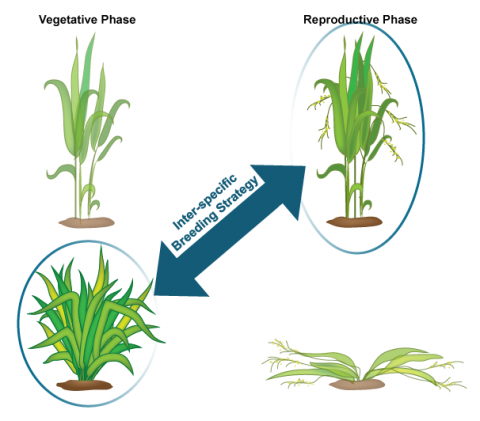
Biology of the Crop
General Characteristics of the Development of the Rice Plant
The growth of rice plant can be divided into three developmental stages (Fig. 6):
- vegetative (germination to panicle initiation)
- reproductive (panicle initiation to heading);
- grain filling and ripening or maturation (milky stage to maturity)
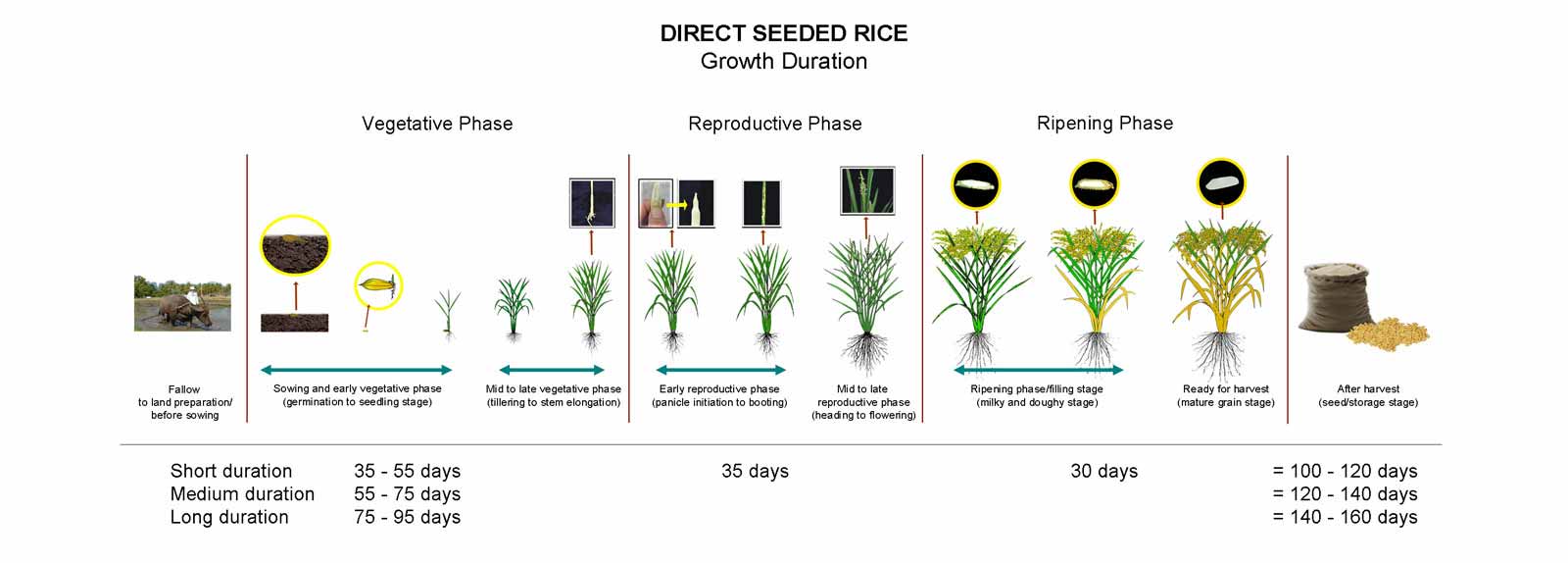
Development Stages
The vegetative phase is subdivided into three stages
- Germination
- Early seedling growth and
- Tillering
The reproductive phase is subdivided into four stages
- Stem elongation
- Panicle initiation
- Panicle development
- Flowering
The ripening phase is subdivided into four stages
- Milk grain
- Dough grain
- Mature grain
In a tropical environment, approximately half of the days of growth (from seeding to harvest) are in vegetative phase, and one-quarter each in the vegetative phase and the ripening phase.
Seed Development
In 2000, Counce et al., proposed a rice developmental staging system divided into three main phases of development:
- Seedling
- Vegetative
- Reproductive and Ripening
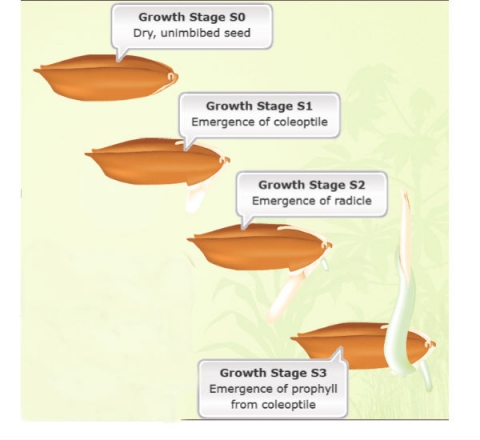
The sequence of normally occurring seedling developmental events is presented, noting that there are exceptions to the sequence given.
Seedling development can be further divided into four stages (Fig. 7):
- Unimbibed seed (S0)
- Coleoptile emergence (S1)
- Radicle emergence (S2)
- Prophyll emergence from the coleoptile (S3)
Vegetative Development
Vegetative development consists of V1, V2…… VN; where N is equal to the final number of leaves with collars on the main stem (Fig. 8).
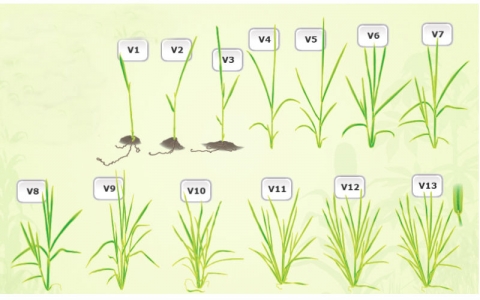
Reproductive Development
Reproductive development consists of 10 growth stages based on distinct morphological measures as shown in Table 9.
R0
Panicle development has initiated |
R1
Panicle branches have formed |
R2
Flag leaf collar formation |
R3
Panicle exertion from boot, tip of panicle above collar of flag leaf |
![]() |
![]() |
![]() |
![]() |
R4 | R5 | R6 | R7 |
One or more florets on the main stem panicle has reached anthesis | At least one caryopsis on the main stem panicle is elongating to the end of the hull | At least one caryopsis on the main stem panicle has elongated to the end of the hull | At least one grain on the main stem panicle has a yellow hull |
![]() |
![]() |
![]() |
![]() |
R8 | R9 | ||
At least one grain on the main stem panicle has a brown hull. The brown hull indicates the grain has begun to dry. | All grains which reached R6 have brown hulls | ||
![]() |
![]() |
Further Characteristics
The grain yield of rice is comprised of the following four components:
- Number of panicles/m2
- Number of grains / panicle
- Percentage of ripened grains
- 1000 gm weight.
However, plot yield weight remains the best way to determine the yield of lines or genotypes.
Photosynthesis
Rice is a C3 plant. C3 photosynthetic pathway is not very efficient at transforming inputs to grain, in comparison to the C4 pathway. In order to increase rice yield there are ongoing efforts on development of C4 rice to create a new type of rice with enhanced photosynthetic capacity (Susanne von Caemmerer et al, 2012). The effort to develop C4 rice is worth it as rice is the staple food source in many Asian countries like India, China and Japan, and also it is grown in places where maize cannot be grown.
Photoperiod and Temperature
Oryza sativa is classified as a short-day plant (i.e., requires long nights to flower). This means that heading date (the number of days it takes for the panicle to begin to exert from the boot, that is, the flag leaf sheath) is accelerated under short-day conditions, while heading date is delays when long-day conditions exist (Garner and Allard, 1920).
General Classification of Rice Production Systems
According to the International Rice Research Institute (IRRI), rice can be classified into four major production ecosystems (Fig. 10):
- Irrigated rice – Rice is grown in well watered condition and is flooded throughout the rice growing season.
- Rainfed lowland rice – Rice grown under this condition is dependent on rainfall only, and land is prepared such that it preserves the rain water.
- Upland rice – Rice grown without irrigation water and relies completely on rainfall.
- Flood-prone rice – Rice grown in river areas is deep water rice, with no inbuilt water control system.
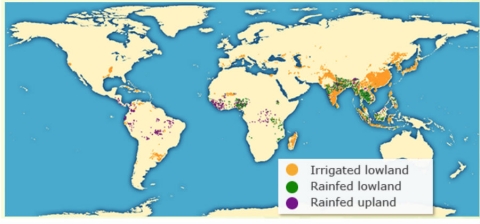
Rice Ecosystem | Total Production area (%) | Total rice production (%) |
Irrigated lowland | 55-60 | ≈ 75 |
Rainfed lowland | ≈ 30 | ≈ 20 |
Rainfed upland | ≈ 10 | < 5 |
Diversity
There is huge diversity in Oryza species for shape, color, size as can be seen in Fig. 11. Rice production totals in different regions of the world is shown in Fig 12.
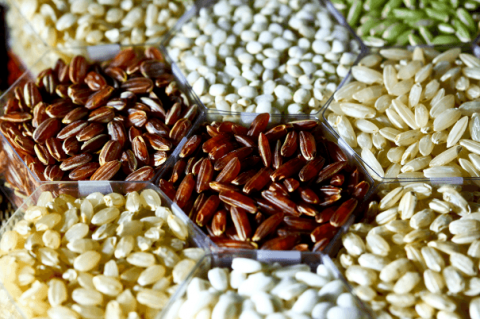
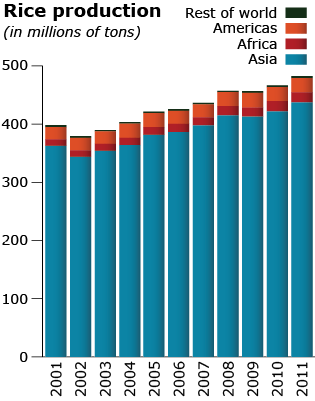
Adaptation, Economic Importance and Uses
Rice in the Human Diet and Nutrition
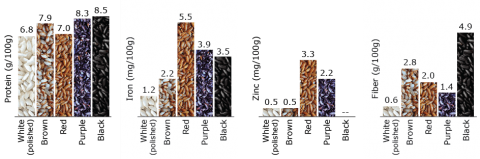
Processing
The procedure of milling and consequently polishing rice, results in the highly valued white rice which removes nearly all the outer layers and germ and leaves a product deficient in thiamine. Through fortification and parboiling, adequate quantities of thiamine and other B vitamins can be retained in rice. Parboiling is usually done in the mill where unhusked rice is generally steamed, so that water is absorbed by the whole grain providing an even distribution of vitamins in the whole grain. However, in conventional methods, the paddy is dried and dehusked prior to milling.
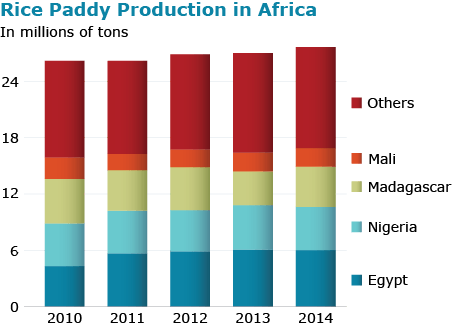
International Breeding Centers
The Consultative Group on International Agricultural Research (CGIAR) has three centers:
- The International Rice Research Institute (IRRI) has a global mandate to work on rice and its headquarters is in Los Baños, Laguna in the Philippines.
- The West Africa Rice Development Association (WARDA) has mandate to work on rice in West Africa.
- The International Centre for Tropical Agriculture (CIAT) has the regional mandate to work on rice in Latin America.
Breeding Methods
Heterosis
Heterosis refers to the superiority of the F1 hybrids resulting from a cross of diverse parents, over their parents in performance of desired traits, for example, vigor, yield, number of productive tillers, panicle size, number of spikelets per panicle.
The crosses (×) between rice subspecies showing heterosis in decreasing order are as follows (Fig. 14):
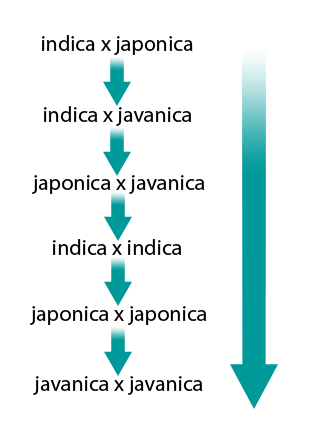
Types of Heterosis
Intrasubspecific heterosis: It is the most commonly used heterosis which provides around 15% to 20% more yield than the best check grown under similar conditions. For example
- indica × indica
- japonica × japonica
Intersubspecific heterosis:Oryza sativa ssp. indica and ssp. japonica are two of the most common subspecies of cultivated rice. The cross between these two subspecies shows maximum heterosis in the F1 hybrid. Major limitation in intersubspecific heterosis is the high spikelet sterility and long growth duration. The discovery of wide compatibility (WC) genes has provided a solution to overcome these problems allowing the utilization of these type of crosses.
Interspecific heterosis: The crosses in cultivated species refer to only O. sativa and O. glaberrima. However, heterosis of yield is very high and plant stature remains a problem.
In rice, the interspecific F1 hybrids cannot be used commercially. Examples of interspecific hybrids as a result of wide hybridization, generates genetic variability and bring together several biotic and abiotic stress resistance genes.
- O. sativa × O. longistaminata
- O. sativa × O. rufipogon
- O. sativa × O. perennis
Hybrid Rice
For details on hybrid rice research at public and private commercial sectors, refer to the following links:
The commercial rice crop grown as a hybrid crop is an F1 hybrid developed from the cross of two genetically diverse pureline parents. Good rice hybrids have the potential to yield 15-20% higher than the best pureline cultivar when these two (hybrid and pureline parents) are grown under similar conditions.
Since rice is a self-pollinated crop, the male sterility system has been used to develop commercial rice hybrids. Commercial companies are more interested in developing hybrid cultivars because of the profits accrued from farmers returning each year to buy new seed. The higher cost of hybrid seed is partly due to the increased cost of development of the parents used to make the hybrids.
Male Sterility in Rice
Male sterility is defined as the inability of a plant to produce functional pollen grains. The use of male sterility in hybrid seed production has a great importance as it eliminates the process of mechanical emasculation. Three forms of male sterility that can be used are:
- Cytoplasmic genetic male sterility (CGMS)
- Environment-sensitive genic male sterility (EGMS)
EGMS is classified in the following categories- TGMS: temperature-sensitive genetic male sterility
- rTGMS: reverse temperature-sensitive genetic male sterility
- PGMS: photoperiod-sensitive genetic male sterility
- rPGMS: reverse photoperiod-sensitive genetic male sterility
- PTGMS: photothermosensitive genetic male sterility
- Chemically induced male sterility (CIMS)
Cytoplasmic Genetic Male Sterility
In CGMS, three lines are involved in hybrid rice development and the process flow is as follows (Fig. 15):
- Cytoplasmic male sterile line (A line) – The male sterility is controlled by the interaction of sterile cytoplasm (S) and fertility-restoring genes (rf) present in the recessive form in the nucleus.
- Cytoplasmic male fertile line also known as maintainer (B line) – is iso-cytoplasmic to the CMS A-line since it is similar to it for nuclear genes but differs as it has normal cytoplasmic factor (N). A-lines are developed from B-lines using backcross breeding to transfer the CMS gene. The N gene makes the B-line self-fertile, and is used in crossing with the sterile A-line to maintain A-line seed production.
- Restorer line (R line) – R line possesses dominant fertility-restoring genes (Rf), and so is different from the A line and B-line; the restorer line is developed separately to maintain genetic dissimilarity from A-line for expression of heterosis. The restorer gene in the dominant homozygous (RfRf) or heterozygous (Rfrf) state has the ability to restore the fertility in the F1 hybrid.
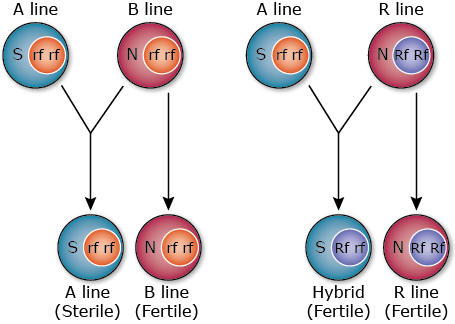
Hybrid Seed Production Using CGMS
Hybrid seed production involves two steps:
1. CMS line (A line) multiplication (AxB): The seed of the CMS line (A line) is multiplied by crossing with the maintainer line (B line) either by hand (in plant breeding programs where a small quantity of seed is required) or in the field under isolation by space or time (to produce breeder seed for commercial seed production). Remember that the A-line is only a parent in hybrid production and it is not for commercial seed sale for farmer production. Seed companies may be interested to obtain marketing rights of A-line for their hybrid breeding program). Generally, A-line seed production field consists of 6 or 8 rows of A-lines alternating with 2 rows of B-lines. This pattern is repeated throughout the field as depicted below (6 rows of A-lines, alternating with two rows of B-lines and repeating pattern in field).
… B B A A A A A A B B A A A A A A B B A A A A A A B B …
2. Hybrid seed production (AxR): Hybrid seed is produced by crossing the A line with the R line in isolation. The hybrid seed is sold to farmers for commercial production so a large increase of parent seed and hence hybrid seed is necessary. More than one location may be planted to make the hybrid seed to minimize the impact of loss of field due to an environmental event or other causes. In the field, 8-10 rows of A-lines are grown interspersed with two rows of R-line to produce hybrid seed.
… R R A A A A A A A A R R A A A A A A A A R R A A A A A A A A R R …
Additional Information For Hybrid Seed Production
- The planting dates may need to be staggered to achieve synchronized flowering of the two parents.
- For better pollen dispersal (from male parent) and seed set (on female parent), ropes or sticks are often used.
- Hormone treatment, such as Gibberellic acid (GA), can increase the receptivity of female to accept pollen. This happens due to better emergence of female panicles from the sheath, exposing the ovary to male pollen.
Hybrid Rice Production Flowchart
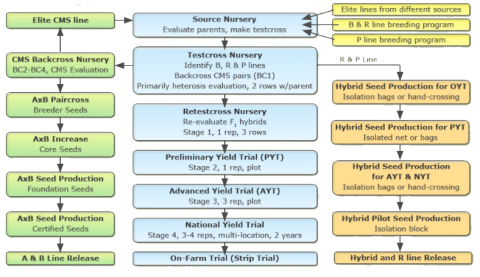
Hybrid Rice Breeding using EGMS
In the two-line method, the male sterile line is (male sterility controlled by a recessive gene) crossed to a pureline that is male fertile (i.e. it possesses the dominant gene for sterility). Male sterility in the female line is genetically controlled by recessive genes and sterility expression is influenced by environment (temperature, photoperiod, or both), and the male parent is selected to be good pureline pollen producer.
Classification of the EGMS System
Types of EGMS Systems Used in Two-Line Hybrid Rice Breeding
- Thermo-sensitive genetic male sterility (TGMS) – In TGMS lines, the sterility or fertility expression is controlled by temperature. Regardless of photoperiod, TGMS lines are usually highly sterile under high temperature and highly fertile under low temperature. The first TGMS line was reported by Japanese Scientist in the rice variety Remei where gamma ray induced mutation resulted in sterility (31-24ºC) to partial fertility (28-21ºC) and complete fertility (25-15ºC).
- Photoperiod-sensitive genetic male sterility (PGMS) – In PGMS lines, the sterility or fertility expression is controlled by daylength. Under long-day conditions, most PGMS lines remain male sterile. Under short-day conditions, they revert back to being fertile. The first spontaneous PGMS mutant, Nongken 58S (NK58S), was reported in 1973 from the japonica (O. sativa ssp. japonica) cultivar Nongken 58 (NK58). NK58S retained male sterility under long day length (longer than 13.75 h) during anther development, while under short day length (less than 13.5 h), partial or complete male fertility was observed. Temperature response was also observed for this line. Under long-day conditions at high temperatures (~29°C) slightly more male sterility was observed.
- Reverse photoperiod-sensitive genic male sterility (rPGMS) – PGMS lines express sterility under short day length and under long day length revert to being fertile. This system is known as reverse PGMS (rPGMS).
- Photo-thermosensitive genetic male sterility (PTGMS) – PTGMS lines are sensitive to both photoperiod and temperature. Temperature is the important factor since PTGMS lines become completely male sterile or fertile beyond (over or under) a threshold temperature range, without any influence of photoperiod. In this system the effect of temperature and photoperiod is difficult to separate and under natural conditions both factors interact to determine sterility or fertility.
Advantages of EGMS Systems
- There is no requirement for seed multiplication of a maintainer line, therefore making the seed production system cheaper.
- No need for backcross breeding to develop a CMS A-line from B-lines.
- Hybrid breeding efficiency is higher in two-line breeding than three-line breeding since it allows use of any fertile line as a pollen source parent.
- Undesirable effects of sterility-inducing cytoplasm do not occur.
- It is ideal for developing indica by japonica hybrids as there is no requirement for restorer lines.
Disadvantages of the EGMS Systems
- The sterility trait is under the control of environmental factors, and any variation such as temperature fluctuation because of a storm, rain etc., will impact the sterility of EGMS lines.
- Seed production can be done in the latitudes with optimal photoperiod length, therefore limiting options in some cases for which locations can be used. The seed multiplication (lines and hybrids) are constrained by space and season.
Two-Line System Production Flowchart (Fig. 17)
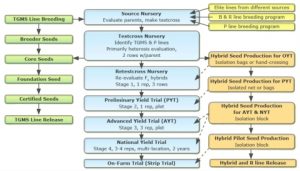
Conventional Rice Breeding Program
In conventional breeding, and for rice programs as well, two parents are crossed and segregating generations are screened for the trait of interest, for example, disease resistance, maturity, height and protein. Uniform lines are tested for yield and along with resistance, desirable varieties are selected and released. The development process from making the initial cross to variety release is shown in Fig. 18.
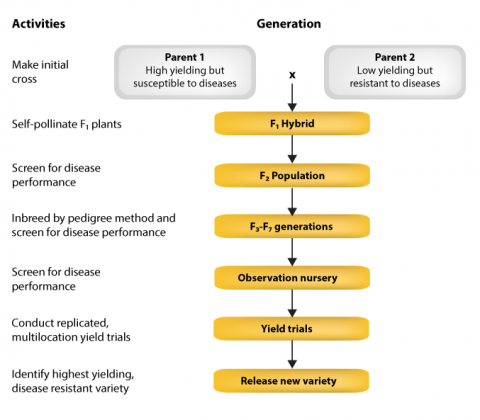
References
Counce, P. A., T. C Keisling, and A. J. Mitchell. 2000. A Uniform, Objective, and Adaptive System for Expressing Rice Development. Crop Sci. 40: 436-443.
Dingkuhn, M., B.B. Singh, B. Clerget, J. Chantereau, and B. Sultan. 2004. Past, Present and Future Criteria to Breed Crops for Water-Limited Environments in West Africa. In Fischer T et al. New directions for a diverse planet: Proceedings for the 4th International Crop Science Congress, Brisbane, Australia, 26 September – 1 October 2004.
FAO 2014. Rice Market Production Monitor, April 2014.
Garner, W. W., and H. A. Allard. 1920. Effect of the Relative Length Of Day And Night And Other Factors Of The Environment On Growth And Reproduction In Plants. Mon. Wea. Rev., 48, 415–415.
Harris, D. R. 1996. The Origins and Spread of Agriculture and Pastoralism in Eurasia. Psychology Press. p. 565.
Huang, X., N. Kurata, X. Wei, Zi-Xuan Wang, A. Wang, Q. Zhao, Y. Zhao, K. Liu, et al. 2012. “A map of rice genome variation reveals the origin of cultivated rice”. Nature 490 (7421): 497–501.
IRRI. 2009. Rice growing environments, Lesson 1: Where is rice grown? http://www.knowledgebank.irri.org/submergedsoils/index.php/rice-growing-….
IRRI. World Rice Statistics Online Query Facility. http://ricestat.irri.org:8080/wrs2/entrypoint.htm.
Khush, G. S. 2001. Green revolution: the way forward. Nature Reviews Genetics 2, 815-822. Kovach MJ, Sweeney MT, McCouch SR. 2007. New insights into the history of rice domestication. Trends in Genetics 23:578-587.
Linares, O. F. 2002, African rice (Oryza glaberrima): History and Future Potential. PNAS 99 (25):16360-16365.
Londo J. P., Y.C. Chiang, K. H. Hung, T. Y. Chiang, and B. A. Schaal. 2006. “Phylogeography of Asian wild rice, Oryza rufipogon, reveals multiple independent domestications of cultivated rice, Oryza sativa”. Proc. Natl. Acad. Sci. U.S.A. 103 (25): 9578–83.
Molina, J., M. Sikora, N. Garud, J. M. Flowers, S. Rubinstein, A. Reynolds, P. Huang, S. Jackson, B. A. Schaal, C. D. Bustamante, A. R. Boyko, and M. D. Purugganan. 2011. “Molecular evidence for a single evolutionary origin of domesticated rice”. Proceedings of the National Academy of Sciences 108 (20): 8351.
von Caemmerer S., W. Paul Quick, and R. T. Furbank. 2012. The Development of C4 Rice: Current Progress and Future Challenges. Science 336, 1671-1672.
Vaughan, D.A., B. Lu, and N. Tomooka. 2008. “The evolving story of rice evolution”. Plant Science 174 (4): 394–408.