Chapter 5: Steps in Cultivar Development
Asheesh Singh; Arti Singh; and Anthony A. Mahama
Cultivar development is one of the four plant breeding projects (other three are genetic improvement, trait integrations and product placement) of many breeding programs. The end product depends on the specific objectives, the mode of propagation and commercial production, and like most other processes, specific steps need to be followed in succession (in parallel in certain cases) to ensure the set objectives are met.
- Describe the basic steps in the development of clonal, inbred, synthetic, hybrid, multilines, and blended cultivars
- Distinguish among the different clonal types and source of variation
- Know the application of male sterility in hybrid crop development
Asexually Propagated or Clonal Cultivars
In Chapter 1, we looked at different types of cultivars that are grown by farmers. These cultivars may be sexually or asexually propagated. Clones are types of cultivars that are asexually propagated. We also learned in this module the concept of heterogeneous versus homogenous and heterozygous versus homozygous. Clonal cultivars are heterozygous and homogenous and can be maintained through vegetative plant parts. Figure 1 depicts the gradation of heterogeneity and heterozygosity in different types of varieties.
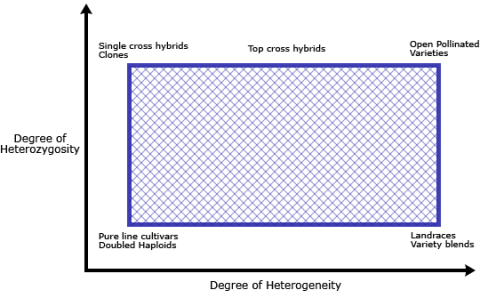
Clones
Clones, as the name implies, are identical copies of a genotype. A population of clones of the same genotype is homogeneous (since they are identical). However, individually, they are highly heterozygous. Asexually or clonally propagated plants produce genetically identical progeny. Since the cultivar can be asexually propagated, heterosis can be fixed as long as the propagation continues. Clones can be the product of inter-generic or inter-specific crosses because even sterile hybrids can be maintained in clonally propagated crops.
One of the biggest challenges in clonal crops production is to keep parental lines and breeding stocks free of virus and other disease that can be transmitted through vegetative propagation.
In-vitro methods (such as, tissue culture) are often used to rapidly increase clonal stocks which can be kept disease free. The tissue culture methods of plant propagation, known as micropropagation, utilizes the culturing of apical shoots, axillary buds and meristems on sterile suitable nutrient medium to grow new clones. Micropropagation offers an ability to continually produce clones (all year round), produce and propagate hybrids, and produce disease free plants. It is a cost effective technique and requires small space.
Somaclonal Variation
Clones are products of mitosis. Any variation occurring among them is environmental in origin. Micropropagation or tissue culturing can lead to somaclonal variation (SV). SV can be defined as genetically stable variation generated through plant tissue culture (Larkin and Scowcroft, 1981). It has been used by breeders as an approach to create and exploit greater genetic diversity and provides a mechanism to expand germplasm pool for plant improvement and cultivar development.
While the resultant success of SV has not been as great as initially promised, it has led to identification of valuable genotypes, for example, Aluminum tolerance in rice (Jan et al 1997). SV can also cause negative effects, therefore mechanism of genotype purity is desirable in clonal crops. Whether natural (spontaneous) or artificial (induced), somatic mutations are characterized by tissue mosaicism, called chimerism. In a chimera, an individual consists of two or more genetically different types of cells, i.e. mosaics, which can only be maintained by vegetative propagation (not transferable to progenies by sexual means as clones are products of mitosis and change did not happen in sex cells).
Examples of Clonal Cultivars
Many clonal cultivars are in use. Fig. 2 and Fig.3 show some examples.

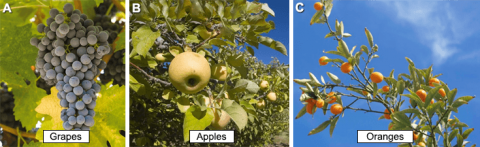
Steps in Development of Clonal Cultivars
Clonal cultivars are developed using the micropropagation method, which involves the following steps.
- Selection and maintenance of stock plants for culture initiation,
- Initiation and establishment of culture – from an explant like shoot tip, on a suitable nutrient medium,
- Multiple shoots formation from the cultured explant,
- Rooting of in vitro developed shoots, and
- Transplanting and hardening, i.e., acclimatization before transplanting to the field.
Breeding Approaches Used In Asexual Crops
Clean (disease-free) clonal material is essential starting material for multiplication for propagation. It is very important in clonal crops to maintain disease-free and/or purify an infected cultivar (Fig. 4). Infection can occur due to bacteria or viruses. Viruses are more damaging due their systemic nature. In order to screen for disease-free material plant material is visually inspected for the presence of pathogens, however this is not the most effective method for viruses as there may be no obvious symptoms.
Detection of Specific Pathogens
Two main methods are used to detect the presence of specific pathogens:
- Serological, such as enzyme-linked immunosorbent assay (ELISA)
- Nucleic acid based, such as Real Time-PCR.
These techniques can detect latent viruses as well as other pathogens. Note that a negative test may not always be proof of the absence of pathogens, and could just be due to ineffective assay.
If diseases or viruses are detected, it is important to eliminate them. Methods used include:
- Tissue culture: Even when the pathogen is systemic, tissue from the terminal growing points can be used for further propagation as it is often pathogen-free.
- Heat treatment: works well for fungal, bacterial, and nematode infections. For viruses, a longer treatment is required relative to other pathogens.
- Chemical treatment: surface sterilization with chemicals can be used to eliminate pathogens.
- Use of apomictic seed
Apomixis
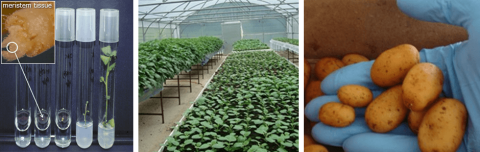
Apomixis is the formation of seeds without meiosis, and two forms are present.
- Gametophytic apomixis in which the asexual embryo is formed from an unfertilized egg; and
- Adventitious embryony, in which the asexual embryo is formed from nucellus tissue.
Steps in Development of Apomictic Cultivars
Apomictically produced seeds are genetically identical to the parent plant. Breeding of apomictic species requires developing population improvement by sexual reproduction and subsequent variety development by apomixis.
Example of plant species in which apomictic cultivar has been produced is Kentucky bluegrass.
Summary of Steps
Step 1: Defining objectives
Step 2: Develop a segregating population
Step 3: Select superior plants (clones)
Step 4: Preparation of seedstock for commercial planting
After objectives are clearly defined and are considered biologically feasible, genetic improvement in clonal crops starts with the assembly and evaluation of a broad germplasm base, followed by production of new recombinant genotypes derived from selected elite clones and careful evaluation in a set of representative environments.
In a crop with few years of breeding efforts, the divergence between landraces and improved germplasm is not as wide as in crops with a more extensive breeding history. As a result, landrace accessions play a more relevant role in clonal crops such as cassava (with fewer years of directed breeding efforts compared to other crops such as potato).
Longer-Term Breeding Experience
With longer term breeding experience, a breeder will have a better understanding and handle on combining ability of different clones as well as more information on other traits such as quality and pest resistance. Thus it is essential to pick parents that will have a higher chance to produce offspring that will be superior and a commercial success. It is important to recognize the difference between botanical (or biological) seed and vegetative (clonal) parts (used for propagation).
The F1 obtained from crossing is biological seed, while the plant parts used from that F1 for further testing in subsequent generations is are clones (identical to the F1 plant).
Several cuttings or plant parts taken for testing from a single F1 will be identical to each other and to the F1, while plant parts taken from different F1 plants will be dissimilar to each other.
In the case of Cassava, the botanical seed obtained after crossing is either planted directly in the field or first germinated in greenhouse conditions and then transplanted to the field when growth is suitable to transplant. Root systems in plants derived from botanical seed and vegetative cuttings may differ considerably in their starch content and therefore selections should not be made for traits that will differ between biological seed and cuttings due to low correlation between trait data from plants developed from biological seed and vegetative cuttings.
Root Data
Since the commercial product is derived from vegetative cutting, root data from biological seed is not relevant for use in selection (unless the correlation is high). One way to overcome this issue is to germinate the biological seed and then transplant, which then develops a root system similar to what will be observed with vegetative cuttings. In clonal crops, the area of plant used to get cutting also influences the performance. Therefore plant breeders should be aware if these are issues in the crop they are working on. In Cassava, vegetative cuttings from the mid-section of the stems usually produce better performing plants than those at the top or the bottom. This variation in the performance of the plant, depending on the physiological status of the vegetative cutting, results in larger experimental errors and undesirable variation in the evaluation process. Consistency in vegetative cutting is important to remove this unwanted source of error. Breeders should also be aware of the number of cuttings that can be obtained per plant as one of the biggest constraints in getting to multi-location trial is the inability to produce enough cuttings per plant to put them into replicated evaluations across several locations.
Crossing Block
Crossing block can be in the field for over a year. In the case of Cassava, synchronization of flowering may require that crossing blocks are maintained for as many as 18 months and on average only one or two seeds per cross can be obtained (in directed crosses). The first selection can be conducted in the third year in the nurseries with plants derived from botanical seed. Due to low correlations between the performance of individual plants and yield plots, selections should be done only on traits with high heritability. Traits with higher heritability can be plant type, branching habits, reaction to diseases. Breeders will be able to reduce their population by up to 60-80%, making the numbers suitable for clonal evaluation trials (generally based on 6-10 vegetative cuttings in Cassava). At this stage, breeders may just use spray paint or tags or another method to identify the discards as some of the traits are visually assessed, and data collection may not be feasible in all cases. From about 100,000 F1 plants a breeder will be able to use single plant selection to reduce the entries going to clonal evaluation trials to about 2,000 to 3,000 clones, where each clone is going to consist of 6-10 plants (coming from vegetative cuttings). In each trial, care is taken to ensure that the same number of plants are grown (for each entry) to avoid bias in selection.
Plot Considerations
As in row crops, plant breeders will use ways to ensure that plots are of the same size (length and width) so that no plot is given unnecessary advantage just because it had a larger area. Either a GPS planter is used so that plots are of the same size, or a trimmer is used to cut the plots to be equal size, or proper measurements are made prior to planting (i.e., if hand planting).
These clones are planted in an un-replicated single row trial generally. Since there is going to be a lot of variability in canopy coverage, height, branching (plant architecture traits), breeders will either try to group more similar entries into same test, or select for high heritability traits (as mentioned above, including harvest index, plant type, branching habit, leaf type, or some other higher heritability quality trait).
To reduce this problem of inter-plot competition, the distance between rows can be increased and plant-plant distance reduced, or leave an empty row between plots. This planting strategy increases the competition among plants from the same genotype and reduces the competition between plants from different genotypes.
It is also advisable to consider dividing fields into smaller blocks and conducting selection within a block (with commercial or elite checks present regularly across the field within each block). It is advisable to record all trait data as it helps a breeder to assess within (all clones from a cross) and among (between different crosses) family performance, and gives an indication of the parents used in crossing.
Trait Assessment
Traits with intermediate to lower heritability can be selected for in the evaluation trials including root or tuber dry matter (which are the economic part and most important breeding objective). The clones keep getting included in advanced trials (from clonal evaluation tests > Preliminary yield tests > advanced yield test > regional tests; number of entries reducing at each stage, vigor of testing increasing in terms of locations, replication, traits) to assess their yield and stability. Also processing and consumer preference (i.e. end-use quality) traits are assessed, and since such traits are most expensive or cumbersome to test, only the most advanced material is tested for these traits. As the most promising material is getting evaluated for processing and end use quality, the breeder needs to start the steps to multiple the stock for commercial planting.
It is generally accepted that at the PYT and AYT stages, testing at more locations will be more beneficial than using more replications, and 2-3 replications per entry should suffice in most cases.
Steps in Development of Self-Pollinated Cultivars
Pure Line Cultivars
Pure line cultivars are developed in self pollinating species. Pure line cultivars are homogeneous and homozygous and, once created, can be maintained indefinitely by selfing. Inbred lines are different from pure lines, although sometimes people use the terms interchangeably.
Inbred lines are developed in cross-pollinated species through inbreeding and these lines are used as parents in the production of hybrid cultivars and synthetic cultivars. Inbred lines are not meant for commercial release to farmers for commercial production because inbred lines suffer from inbreeding depression (yield will be lower than in hybrid and even an open-pollinated variety (OPV). Inbred lines are homogenous and homozygous, similar to pure lines; however, artificial selfing needs to be done at each generation to maintain or increase seed. Sib-mating can be used to avoid severe inbreeding depression.
Summary of Steps in Development of Self-Pollinated Cultivars
Step 1: Define objectives
Step 2: Form the genetic base by creating segregating population(s)
Step 3: Perform selection to make pure lines
Step 4: Conduct Trials (testing of experimental lines) and Seed Multiplication
Clearly Defined Objectives
Step 1: It is critical that a plant breeder has clearly defined objectives before any other activity happens. Objectives need to be clearly defined and biologically possible. Also consider the following when defining objectives:
- Will it meet the needs of the producer, processor and consumer? The best way to accomplish this is by having direct interactions with these three groups. Reading news print and other sources will also give a breeder an indication of the requirements by these groups. If possible, a breeder should attend farm shows, farm group meetings, meet and visit processing companies, colleagues in other disciplines, and marketing companies.
- Available resources. Do you have the necessary resources to achieve the objectives? For example, if you would like to select for resistance to a disease using molecular markers very closely linked to the gene as you don’t have disease nursery available. However, if you don’t have access to either the disease nursery or marker screening lab, it will be near to impossible to meet the objective of developing cultivars with resistance to that disease.
Without clear and logical objectives, a breeder is working aimlessly. It is analogous to driving a car without knowing the destination.
Making Strategic Decisions
Setting objectives allows a breeder to make strategic decisions, such as:
- Picking parents that have the necessary complementation of traits to develop progeny that possesses desirable traits from both parents.
- Which breeding method to use
- Determine selection strategy and plan for any specialized nursery or tools.
- Breeder can also make decisions on which traits to select for and in which generations.
Step 2: Based on the objectives, a breeder can pick the parental material which can be:
- Advanced lines from the breeding program
- Advanced lines from another breeding program
- Released cultivars
- Germplasm line from gene bank or a pre-breeding program
- Introductions (from other countries) from colleagues or genebank
- Mutant lines, populations (unselected or selected)
- Wild relative (need to be crossable or resources available to do embryo rescue if needed)
Crops that have a long history of breeding efforts will rely on cultivars and advanced breeding lines and in specialized cases, introductions as the choice of parent material. Crops with less breeding effort will rely on populations and introductions. Majority of parents in a breeding program will be best lines (advanced lines or newest cultivars) derived from the continuous breeding cycle of the program.
Parents Selection
All the traits desired in the cultivar you want to develop need to be present in the parents. Parents selection has to be based on reliable and complete data (yield testing, adaptation testing, specialized nurseries for stress assessment, end use quality, etc.). If a breeder does not have the specialized nurseries, she/he would collaborate with other breeders in the same or different organizations to send material for testing and characterization (material transfer across organizations may need a material transfer agreement (MTA).
The number of crosses made by a breeding program depends on various factors, such as objectives, resources available, breeding method (determines number of breeding lines that will be generated). In self pollinated crops with pure-line cultivars, it is assumed that the parent seed being used is a pure-line (homogenous and homozygous), but in case a breeder decided to accelerate incorporation of a trait and uses a line that is still segregating visually for a trait, more number of plants will need to be used to increase the probability of recovering desired recombinants. Cross configuration will also dictate how many F1’s to create. If a cross is made between two pure-lines, all F1’s will be heterozygous but homogenous. If a three way cross is made using three different pure-lines, F1’s of the three way cross will be heterozygous and heterogeneous, necessitating large population size of F1’s to be created. There is considerable debate about the relative importance of number of crosses versus population size per cross. In most scenarios there will be an inverse relationship between number of crosses and population size, primarily due to resources available in a breeding program. In their review on this topic, Witcombe and Virk (2001) suggest that the strategy is to make fewer crosses (but very careful decisions need to be made based on prior information and scientific principles to pick parents) that are considered favorable and produce large sized populations from them to increase the probability of recovering superior genotypes.
Appropriate Breeding Method
Step 3: Once the crossing scheme is decided and crosses made, the next stage is to choose an appropriate breeding method to develop inbreeding populations which will be composed of an array of different inbred homozygous lines (pure-lines) where genetic variability exists among but not within lines. All breeding methods in pure-line breeding lead to an increase in homozygosity, a reduction in the genetic variance within families, and an increase in the genetic variance between families. Cultivar development is aimed at identifying the best homozygous lines.
Selection should commence in an early generation and preferably as early as F2 because it is the generation of maximum variability, and the minimum population size required to observe desirable type is lowest in F2 and progressively increases. This means a breeder will not have to evaluate larger populations as the generations advance in order to recover the desirable types.
It is also important that a breeder makes selections in each generation (if possible) so as to continue with the development of pure-lines and to eliminate undesirable types. This may be accomplished through phenotypic or genotypic selection with molecular markers. If a high value marker is, or set of markers are, available and linked to the trait of interest, it will be very beneficial for a breeder to use the marker(s) to enrich and advance the desirable types while eliminating the undesirable types.
Selection Considerations
Consider for example, a trait under recessive gene control, which, in fixed homozygous recessive state, will never segregate to give the desirable dominant allele. Evaluating lines with the undesirable homozygous recessive genotype in this case will be a drain on resources. Therefore, the use of molecular markers (which are not influenced by environment) lends a breeder confidence in the selections made, provided the molecular marker is robust, tightly linked to or is on the gene, and not background dependent.
For selections in early generations, a breeder will likely handle several thousand plants, hills, or rows. It is therefore very important to handle this material in a selection environment that is very similar to the target region or is representative of the target environments of different agro-ecological regions if breeding for a sub-region. In other words ensure that the environment is ideal for selecting for targeted traits. For example, choose dryland environment if breeding for drought tolerance, or irrigated field nurseries if breeding for high-input environments.
It is best to grow these early generation trials at a location where a breeder has easy and quick access to observe the material to facilitate making breeding decisions, and also from a logistical viewpoint, to better manage the trial location.
It is extremely important that a breeder eliminates controllable sources of variation such as weeds, non-uniform land, animal damage, non-uniform application of chemicals.
Generation Trials
In early generations (F2 to F4), selection is restricted to traits of high to moderate heritability, whereas in later generations (F4 to F6 or F8 depending on the complexity of the crop genome), evaluation is more detailed and involves multi-location testing and replication.
In situations of relatively large number of entries but limited resources, in early generation trials, single replication yield plots may be used to identify material to advance. Statistical approaches such as running mean, partial rep or augmented designs can be used to identify promising lines. As generations advance, more seed is available and population sizes are sufficiently reduced to allow for increased replications and locations. Selection is then done for traits of lower heritability, such as yield (in larger or paired rows) and for end use quality. However, techniques that require small sample sizes such near-infrared spectroscopy (NIRS) can be effectively utilized in earlier generation testing of end use quality traits to select and remove the undesirables based on cut-offs developed in comparison with checks or industry requirement. Note that cut-offs for traits are variable in every test as they are generally developed based on checks or industry requirement.
Final Stages
Step 4: Final stages in the breeding cycle will involve lines that are considered pure-lines (non-segregating). At this stage, more extensive testing of few best recombinants from a cross is done for agronomic performance and end-use quality. Multi-environment testing is done for adaptation and stability, and environments may be locations or a combination of locations and years. At this stage, trials will be grown using lattice design (incomplete block if the number of entries is large) or RCBD, and detailed observations made and data taken. Since fewer number of lines is tested, more detailed assessment is feasible for an increased number of traits.
For optimal use of resources and to ensure timely adoption of pure-lines, a breeder needs to pro-actively initiate seed multiplication alongside advanced yield testing for production of sufficient quantities of certified seed for the launch of pure-lines.
In the case of doubled haploid (DH), steps 3 and 4 are very closely aligned and can even be considered as one because once the doubled haploid is generated, lines can go into multiple location replicated testing where seed quantity permits.
Steps in Development of Synthetic Cultivars
Hybrids and Synthetics
Cross-pollinated species have two main types of cultivars: Hybrids and Synthetics.
Hybrids are generally a product of a single cross (or two way cross; A x B), and to a much smaller extent three-way crosses (A x B) x (C) or double-crosses (A x B) x (C x D).
Synthetic cultivars consist of a mixture of heterogenous and heterozygous individuals (parental lines are generally clones or inbred lines). Synthetics are more common in some developing countries. These parental lines are maintained so that synthetic cultivars can be re-constituted when needed. These parental lines (clones of inbreds) are assessed for their general combining ability and lines exhibiting superior combining ability are crossed in a polycross configuration to produce S0. The S0 plants are allowed to intermate to produce S1, which, in the case of asexual propagated crops such as alfalfa, can be sold as a synthetic cultivar. Sometimes S2 may be sold as commercial cultivar but maximum heterosis is observed in S1 and is therefore a more favorable generation for cultivar development. In annual crops such as maize, asexual propagation is not feasible therefore the progression from Breeder Seed to Foundation Seed to Certified Seed production is done from S2 to S3 to S4, respectively.
A synthetic cultivar differs from open-pollinated variety (developed by mass selection). A cultivar developed by mass selection is made up of genotypes bulked together without having undergone preliminary testing to determine their combining ability. This makes an open-pollinated cultivar the same as a landrace cultivar.
Synthetic Cultivars
Hybrids are preferred over synthetic cultivars in crops where hybrids can be created economically and commercialized. However, in crops that show heterosis but hybrid production is difficult, synthetics are important and preferred. Synthetic varieties are known for their hybrid vigour and for their ability to produce usable seed for succeeding seasons. Because of these advantages, synthetic varieties have become increasingly favored in the cultivation of many species, for examples forage crops such as alfalfa.
Summary of Steps in Development of Synthetic Cultivars
- Step 1: Define objectives
- Step 2: Form the genetic base by creating segregating population(s)
- Step 3: Perform selection to make pure lines
- Step 4: Conduct Trials (testing of experimental lines) and Seed Multiplication
We have already covered in details how to set up objectives. Similar principle can be applied to synthetic cultivars to set reasonable synthetics.
Assembly of Parental Lines
Assembly of parental lines can be from a previous synthetic cultivar or from other experimental populations. Parental lines can consist of different clones (forages) or inbred lines (maize). Clones will be highly heretogenous and heterozygous and each clone will be unique. These clonal lines are used to establish a source nursery with several thousand individual plants generally grown in a space planted grid system to reduce environmental variance and enable meaningful comparisons of experimental clones amongst each other or to a check within smaller grids. A breeder will select for higher heritability traits such as disease reaction, and morphological traits. Once the superior clones are identified, they are grown in a polycross nursery to either facilitate random pollination among clones or carefully set up clones to facilitate equal chance per clone to contribute pollen to other clones. Clones may be replicated to ensure uniform pollination. Further evaluation may be done, and seed is harvested (in equal amount per clone per replication). In a perennial species, clones may be grown for more than one year and seed harvest each year. This polycross nursery is used to produce seed for progeny testing in performance tests (in corn, one year but several locations and can be replicated; in forages and perennials, one or more years in several locations and can be replicated). Based on the progeny testing, superior clones from the polycross nursery are identified and crossed to each other to produce the synthetic. Syn1 or Syn2 may be released as a synthetic cultivar in a forage species (with clonal propagation). While in maize, two or three more rounds of pollination may be needed to have sufficient seed for commercial launch of a synthetic cultivar.
Half-sib Selection
Half-sib selection is widely used for breeding perennial forage grasses and legumes. A polycross mating system is used to generate the half-sib families from selected clones maintained vegetatively. The families are evaluated in replicated rows for 2-3 years. Selecting of traits with high heritability, e.g. oil and protein content in maize is effective.
Full-sib mating involves the crossing of pairs of plants from a population in which case control is exerted on both male and female parents.
A half sib is a plant (or family of plants) with a common but unknown pollen parent (i.e. pollen source). Therefore, in half-sib mating, the pollen source is random from the population, but the female plants are identifiable. Half-sib selection is based on maternal plant selection without pollen control, therefore half-sib selection is less effective for changing traits with low heritability. The methods used by plant breeders in population improvement may be categorized into two groups: one group is based on phenotypic selection alone (no progeny testing), and the second group is based on genotypic selection (with progeny testing).
The specific methods include:
- Simple recurrent selection or mass selection. The procedure does not involve the use of a tester so there is no estimation of general or specific combining ability. Selection is based on phenotypic observations and therefore this method is also known as phenotypic recurrent selection.
- Recurrent selection for general combining ability. In this method a wide genetic base cultivar (i.e., a population) is used as a tester to cross with identified females, therefore deploying a half-sib progeny test procedure. Based on the test cross progeny performance in replicated or multi-environment trials, selections are made. Selected lines are advanced into the next round of testing. Generations may be advanced by sib-mating while the progeny test is on-going.
- Recurrent selection for specific combining ability. In this method a narrow genetic base line (i.e., inbred line) is used as a tester to cross with females, also deploying a half-sib progeny test procedure. Similarly, based on the test cross progeny performance in replicated or multi-environment trials, selections are made. Selected lines are advanced into the next round of testing and here also, generation may be advanced by sib-mating while the progeny test is on-going.
- Reciprocal recurrent selection. This scheme is capable of exploiting both general and specific combining ability. This is achieved by using two heterozygous populations, where each population serves as a tester for the other.
[Note: The difference between a synthetic and an open pollinated cultivar is the ability to re-constitute the seed in a synthetic because the parents (inbred, clones, hybrids) are used in a pre-determined manner and configuration, while in an OPV the original population cannot be created due to no control on parent configuration. After a limited number of generations, seed needs to be reconstituted for a synthetic using the breeder selected parental stock, while in OPV the random mating happens in each generation and population can be propogated indefinitely]
Steps in Development of Hybrid Cultivars
Hybrid Cultivars
Hybrid maize (Zea mays L.) in USA is an example of a success story in cross-pollinated crops. Up until the 1930s, open pollinated varieties were the more common type of cultivars in cross-pollinated crops. However since then in USA almost all commercial maize cultivars are hybrids.
A hybrid cultivar is the F1 offspring of a planned cross between inbred lines, cultivars, clones, or populations. The hybrids may be the product of a single cross, a three-way cross, or a double cross hybrid. One absolute requirement for a hybrid cultivar is its superior performance over the parents (heterosis) and an ability to economically generate the seed for commercial seed sale. In the case of maize, farmers moved from OPV to hybrids due to several advantages offered by hybrids which include higher yield, improved tolerance to stalk lodging (i.e. better standability), and improved response to drought. Development of hybrids in maize was easier due to the ability to follow a configuration of males and females for seed production – female rows were detasseled and seed was only collected from female rows (in later years, male rows destroyed post-pollination to ensure no contamination). Therefore, the role of male rows is to serve as pollen source, with requirements that the male needs to be a good combiner to the female (higher SCA) and must possess good pollen shed, nicking well with the female, i.e, must have close flowering time to that of the female.
Cytoplasmic Male Sterility
Discovery of cytoplasmic male sterility (Fig. 5) has also helped in the development of hybrid crops in those species. Plants with sterile cytoplasm plus nuclear non-restorer genes are male sterile; plants with sterile cytoplasm plus nuclear restorer genes produce fertile pollen (fertility restoration). In contrast to plants with sterile cytoplasm, plants with normal cytoplasm are male fertile when they carry either of the nuclear genes: restorer or non-restorer. Fertility restoration may not be needed for crop species in which the vegetative part is of economic value. In addition to straight crossing (male on female) and male sterility, chemical agents have been used to create hybrid cultivars. An important consideration of hybrid cultivars is the expression of heterosis and efficiency (i.e., cost, labor, time) of seed production.
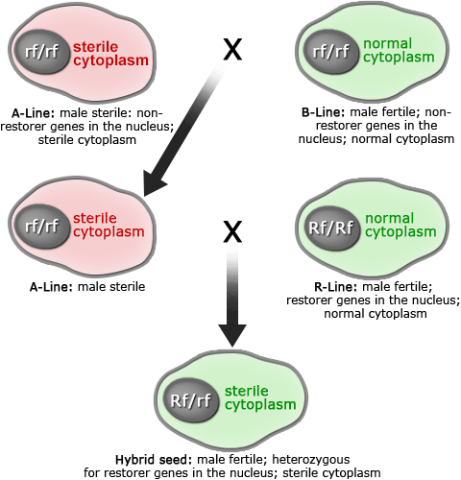
Practical Considerations
Some practical considerations for hybrid systems:
- Farm production of field crops such as wheat, barley, rye, sunflower, grain sorghum requires large amounts of seed for planting the crop and return per unit area is relatively low compared to most horticultural crops or oilseed crops (such as canola), so expensive labor intensive methods of producing hybrid seed (such as hand emasculation) are not preferred. Cytoplasmic male sterility is one approach that is useful in field crops to develop hybridization techniques in which seed parents and pollinators could be grown on a field scale.
- Pollination also needs to be on a field scale, such as with wind or with bees. Successful hybridization via wind pollination requires that males shed abundant amounts of pollen and female lines be receptive (e.g. florets should open at appropriate times). There is a need to synchronous flowering times between females and males.
- Female inbred line should generally be more productive and with a higher capacity to produce more seed. Male and female need to be chosen for their superior specific combining ability, and are generally distinct i.e. belonging to different heterotic groups as for example stiff stalk and non-stiff stalk in maize in North America, or origins as for example indica and japonica in rice.
- Expression of heterosis needs to be sufficient to overcome the cost of development and hybrid seed production. For example, in wheat heterosis is not sufficient to warrant development of a hybrid. Floral morphology also prevents easy pollinations.
Hybrid Rice Breeding
A good example in hybrid rice breeding in China was produced by the International Food Policy Research Institute (Li, Xin, & Yuan, 2009).
History of hybrid rice technological development in China
1964 – Research on three-line hybrid rice initiated
1970 – Wild abortive (WA) rice identified on Hainan Island in China
1973 – Photo-thermosensitive genic male sterile (PTGMS) material identified
1974 – First sets of three lines (A, B and R lines) developed for three-line system hybrid rice
1976 – Hybrid rice commercialization started
1977 – Systematic hybrid rice seed production technique developed
1983 – Hybrid rice seed yield more than 1.2 ton/ha
1987 – Hybrid rice seed yield more than 2 ton/ha
Hybrid rice area more than 10 million ha
National Two-line System Hybrid Rice Program established
1990 – Hybrid rice area more than 15 million ha
1995 – Two-line hybrid rice system developed
1996 – “Super Rice Breeding” national program initiated
1998 – Hybrid rice seed yield more than 2.5 ton/ha
2000 – Super hybrid rice Phase I objective (10.5 ton/ha) achieved
2004 – Super hybrid rice Phase II objective (12.0 ton/ha) achieved
2006 – Super hybrid rice Phase III objective (13.5 ton/ha) initiated
Three-line System
The three-line system (Fig. 6) includes the following lines:
- Male sterile line (A line): The cytoplasmic male sterility trait is controlled by both cytoplasm and nucleus; this line is used as female in hybrid seed production.
- Maintainer line (B line): This line is used as a pollinator to maintain the male sterility. The maintainer line has viable pollen grains and sets normal seed.
- Restorer line (R line): Any rice cultivar that restores fertility in the F1 when it is crossed to a CMS line.
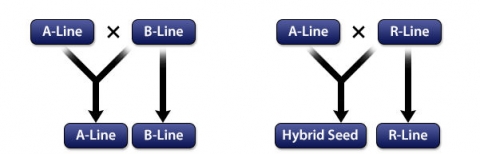
Rice hybrids made with cytoplasmic nuclear male sterility have been grown for several decades in both developed and developing nations. Traditionally, three-line system was used to produce hybrid rice, but more recently an innovative kind of genetic male sterility has been used as well, to make ‘two-line’ hybrid rice. Seed is produced on female inbred lines that are homozygous for environmentally sensitive (photoperiod or temperature or both) recessive male sterility genes. Seed production fields are planted in an environment (e.g. long day and/or high temperature) that enables expression of the male sterility gene in the female and enabling successful hybrid seed production. Seed increase fields of the female lines are grown in an environment (e.g. short day and/or cooler temperatures) that represses expression of the male sterility genes, allowing the female lines to reproduce via self-pollination.
Two-line System
Two-line system hybrid rice included the following two lines (Fig. 7):
- Male sterile line: nuclear gene(s) and environmental conditions such as photoperiod and/or temperature control male sterility. Male sterile lines can be environmental-conditioned genic male sterile (EGMS), photoperiod-sensitive genic male sterile (PGMS), thermo-sensitive genic male sterile (TGMs) or photoperiod- and thermo-sensitive genic male sterile (PTGMS) lines
- Restorer line (R line): any rice cultivar that restores fertility in the F1 when it is crossed to the male sterile line.
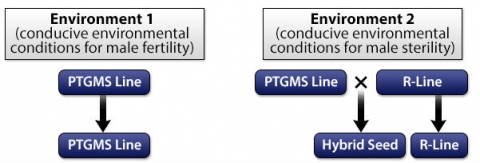
Two-line system has several advantages over three-line system:
- It is simpler as the need of maintainer is removed,
- It is more applicable in diverse genetic background and easier to implement,
- It has reduced cost of breeding program and seed production,
- There is no detrimental effect of CMS system.
However, due to the dependency of trait expression to environmental conditions, problems may arise in hybrid seed production. An important requirement is that environments be chosen that have more consistent temperature and day length at critical times of crop growth.
Hybrid Maize Cultivars
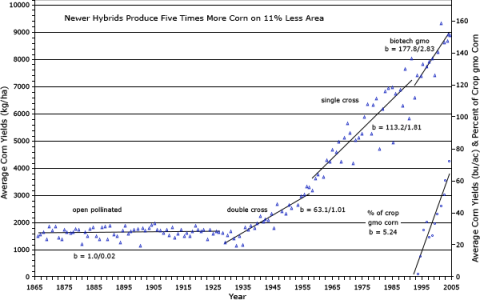
Summary of Steps in Development of Hybrid Maize Cultivars
- Step 1: Development of inbred lines
- Step 2: Making the crosses to produce a hybrid
- Step 3: Testing the hybrids
- Step 4: Development of seed for commercial production
Inbred Lines
Inbred lines can be developed by inbreeding selected heterozygous plants until sufficient homozygosity is reached without severe inbreeding depression. Sib-mating may be used to maintain inbred lines. With the advent of doubled haploid (DH) technology, this problem is minimized. DH lines provide genetic homozygosity in one generation. Because haploids carry only a single copy of every gene, any gene or genes that have deleterious effects for seed or plant development will have immediate genetic effects to depress or inhibit normal seed or plant development so these plants will be quickly eliminated at the haploid stage. Haploids also provide an advantage of better response to marker assisted selection as markers can be used to identify and select for desirable genes, and upon chromosomal doubling these genes are fixed. This provides an efficient and rapid tool to eliminate unfavorable genes and to enrich favorable genes to improve the genetic pool. DH lines have 100% genetic homozygosity, and the technique significantly reduces the time taken to develop inbred parents for crossing. These DH lines do not show inbreeding depression in the following generations, and in the absence of spontaneous gene mutations or transpositions that may cause certain deleterious influences and segregation, DH lines provide a powerful tool in maize breeding. In the DH process only one round of recombination happens thereby minimizes breakage of desirable linkages, as well as also reduces the chances to break undesirable linkages. Therefore a breeder needs to recognize the need for population size optimum, and so larger population sizes may be useful.
Traditionally, open pollinated varieties of maize were the source of inbred lines. However, as the cultivars moved away from OPV to double crosses, three-way crosses or single crosses, which boosted maize yields (Fig. 8), the source of inbred lines also changed. Recurrent selection programs were also a popular source of inbred lines in public breeding programs, but private programs have moved away from recurrent selection programs as sources of inbred.
Heterotic Groups
In North America, for example, heterotic groups have been developed to classify inbred lines, and modern hybrids are the result of crossing a line from one heterotic group with a line from a different heterotic group (Fig. 9). Classification of heterotic patterns is generally based on several criteria such as pedigree, molecular marker based associations, and performance in hybrid combinations. Most conventional inbred line development involves making crosses within a heterotic group and as the population (within a cross) is advancing, testers belonging to different heterotic groups are used in crosses.
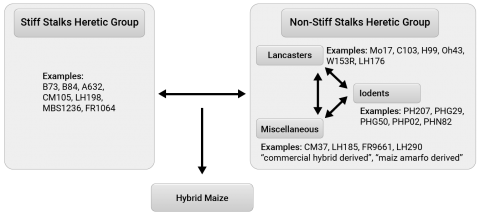
Inbred Line Development
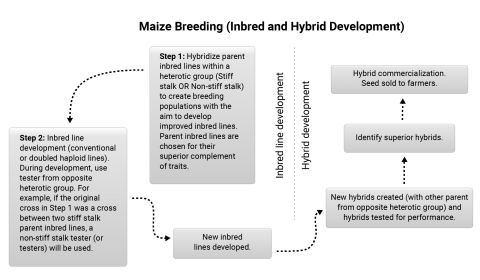
The majority of inbred development activities in North America involve the use of the pedigree method of breeding (Fig. 10) Breeding crosses tend to be made by crossing inbred lines within a heterotic pattern. Inbred lines from the other heterotic patterns are used to improve the heterotic pattern represented by the breeding cross.
Two-parent Cross
Two-parent cross (parents belonging to the same heterotic group) are most common in maize inbred line development. An F2 population is formed from the breeding cross, which is then followed by several rounds of inbreeding using ear-to-row with each family tracing back to different F2 plants. During the inbreeding process, genotypes with obvious defects are eliminated. Early generation testing occurs around the F3 or F4 generation, which involves forming topcross hybrids between the F4 lines and an inbred line from a contrasting heterotic group. This cross can be made in the off-season nursery, and then in the summer (in North America), the resulting topcross hybrids can be tested in two or more environments (Fig. 11). Selection will be based on yield, lodging or stalk strength, maturity, test weight, and height, or other trait of interest. Inbred lines can be advanced in the same season through another round of inbreeding. Lines that produce hybrids considered to have merit (similar or better than commercial checks) are advanced through another round of breeding in the off season nursery and perhaps a round of crossing with inbreds of complementary heterotic group. In the summer, selection is made as described above. At this stage, the superior inbreds are forwarded to hybrid development teams for commercial testing with specific testers and in more environments. [Adapted from: Lee and Tollenaar 2007].
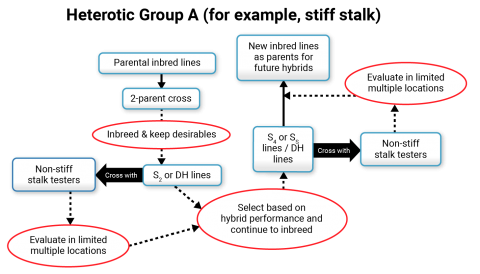
Hybrid Commercialization
In the hybrid development process, more hybrid combinations are tested in fewer environments during the early testing phase, while in the later testing phases fewer hybrid combinations are tested in more environments. Generally, testing involves growing the hybrids in more locations while reducing the number of replications, thus allowing for more vigorous evaluation for adaptation.
Requirements
Several requirements need to be met for inbred lines classified as good parents in hybrid production. For example a female parent must be vigorous and produce high quality, healthy seed, and male parents should produce abundant and good quality pollen. An important consideration for choice of testers in the pedigree type breeding approaches is that testers be from a complementary heterotic group, maximize variance (among test crosses), as well as possess high mean (Bernardo 2010; see chapter 9).
Additional Suggested Web-Based Learning
Steps in Development of Multi-line Cultivars
Multi-lines are generally a set of isolines (traditionally created using backcrossing; or can be through transformation) that differ for one trait or more. These are grown in self-pollinating crops, where cultivars are pure-lines, so a mixture of pure-lines (if they are isolines) can form a multi-line cultivar. This approach has been used to provide control over a prevailing pathogen, such as a multi-line cultivar with different rust resistance genes in wheat. This should theoretically provide better protection against pathogen races and prevent a total crop loss. The pure lines are phenotypically uniform for morphological and other traits of agronomic importance (e.g., height, maturity, photoperiod), in addition to genetic resistance to a specific disease (or any other trait, for example, abiotic stress). See Breth (1976).
Backcrossing is used to develop isogenic lines which are then combined in a predetermined ratio.
Steps in Development of Blends
A blend or composite cultivar, like a multiline, is a mixture of different genotypes. The difference between the two lies primarily in the genetic distance between the components of the mixture. Whereas a multiline consists of closely related lines (isolines), a composite may consist of different types of cultivars. It is intended to pick genotypes in a blend to minimize differences in maturity, growth habit, lodging, and disease resistance in the package. This consideration is critical to having uniformity in the cultivar. However, a different approach would be to pick blends that can be phenotypically different if the intention is to maintain some level of percentages.
WB Seed Company provides additional information to familiarize yourself with examples of a blend cultivar system in commercial production.
References
Bernardo, R. 2010. Breeding for Quantitative Traits in Plants. 2nd ed. Stemma Press, Woodbury, MN.
Breth, S.A. 1976. Multilines: safety in numbers. CIMMYT Today 4.
Breth, J.R., and D.S. Virk. 2001. Number of crosses and population size for participatory and classical plant breeding. Euphytica 122: 451-463.
DePauw, R.M., R.E. Knox, F.R. Clarke, H. Wang, M.R. Fernandez, J.M. Clarke, and T.N. McCaig. 2007.
Shifting undesirable correlations. Euphytica. 157(3): 409-415.
Jan, V.V.S., C.C. de Macedo, J-M. Kinet, and J. Bouharmont. 1997. Selection of Al-resistant plants from a sensitive rice cultivar, using somaclonal variation, in vitro and hydroponic cultures. Euphytica 97: 303310.
Larkin, P.J., and S.C. Scowcroft. 1981. Somaclonal variation-a novel source of variability from cell culture for plant improvement. Theor. Appl. Genet. 60, 197214.
Lee, E.A., and M. Tollenaar. 2007. Physiological basis of successful breeding strategies for maize grain yield. Crop Sci 47(S3):S202-S215.
Li X., Y. Xin, and L. Yuan. 2009. Hybrid Rice Technology Development: Ensuring China’s Food Security. 2020 Vision Initiative. IFPRI publication.
Troyer, AF. 2009. Development of Hybrid Corn and the Seed Corn Industry. In Handbook of Maize Genetics and Genomics (ed). J. Bennetzen and S. Hake.