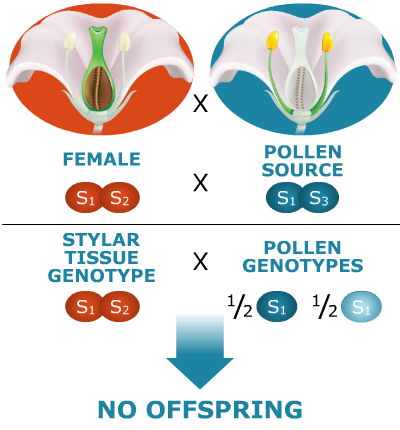
Chapter 3: Controlled Hybridization: Self-incompatibility, Male-sterility, and Sex-inheritance
Deborah Muenchrath; Arden Campbell; Laura Merrick; Thomas Lübberstedt; and Shui-Zhang Fei
Introduction
Most crop species require seed production for their propagation. Some species, however, possess mechanisms that regulate fertility. Such mechanisms can reduce or prevent seed set, and affect self- or cross-pollination. These fertility-regulating mechanisms may be an obstacle or a benefit to the plant breeder. In this module, we’ll explore these mechanisms and their utility.
Learning Objectives
- Mechanisms and utility of incompatibility systems.
- Modes of sex inheritance in plants and their application in plant breeding.
- Male sterility systems and their applications in plant breeding.
Self-incompatibility
Genetic-based Self-incompatibility
Self-incompatibility is the inability of a plant to set seed when self-pollinated, even though it produces viable pollen. In contrast, cross-pollination generally results in seed set in self-incompatible species. Many plant families include species with self-incompatibility systems, such as Fabaceae (Leguminosae), Poaceae (Gramineae), Solanaceae, Brassicaceae (Cruciferae), and Asteraceae (Compositae). Self-incompatibility may be caused by genetic interactions between pistil and pollen, or by physical obstacles that hinder self-fertilization.
Self-incompatibility may be caused by genetic interactions between the pistil and pollen-producing physiological factors that interfere with fertilization of female gametes by male gametes produced on the same plant or on a closely-related plant. Typically one or few self-incompatibility (SI) genes are involved in this self/non-self discrimination process, depending on the plant species. Pollen can be rendered ineffectual at several points in the pollination process:
- Sperm enters the embryo sac but does not fuse with the egg.
- Pollen tube penetrates the stigma but it grows too slowly in the style to reach the ovary while the ovule is still receptive.
- Pollen germinates but the tube is unable to penetrate the stigma.
- Pollen germination on the stigma is inhibited.
Pistil receptivity to a particular pollen grain depends on the SI alleles carried by the pistil. The phenotype of the pollen (its capacity to fertilize the female gamete) is determined by either the pollen’s alleles (gametophytic incompatibility) or by the alleles of the plant that produced the pollen (sporophytic incompatibility).
There are two self-incompatibility systems that result from genetic interactions:
- Gametophytic Self-incompatibility
- Sporophytic Self-incompatibility
Both incompatibility types influence the rate of pollen tube growth, but their genetic controls and location of effect differ. Both types involve multiple alleles.
Gametophytic Self-incompatibility
- Gametophytic self-incompatibility involves the allele possessed by the pollen grain. The incompatibility effect occurs in the style. However, in some species with gametophytic SI, incompatibility is expressed on the surface of stigma (in grasses).
- Gametophytic self-incompatibility is controlled by a series of alleles. The rate of pollen tube growth responds to the allelic interaction of both the style and the pollen.
- If both the stylar tissue and pollen possess identical alleles, pollen tube growth is inhibited.
- If stylar and pollen alleles differ, tube growth occurs at normal rates.
FYI: Homozygous Flowers
What happens if the pistillate flower is homozygous for the S allele?
The same rules apply: if the pollen carries an allele that matches the one possessed by the pistil, fertilization will not occur; if they differ, seed can form. However, it should be noted that plants homozygous for a self-incompatibility allele in the gametophytic system are rare.
Why are plants homozygous for gametophytic self-incompatibility rare?
Homozygotes are unusual because the probability of a pollen grain carrying the same allele as the pistil successfully overcoming the incompatibility of their matching alleles is small—but such events do occur occasionally. Thus, a population may have a few individuals homozygous for an S allele.
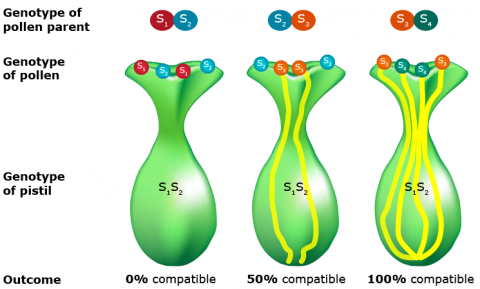
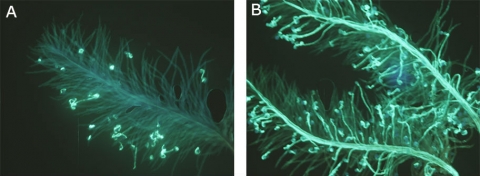
In Fig 2A, all pollen grains are incompatible with the pistil as they fail to germinate beyond the stigmatic surface.
In Fig 2B, nearly all pollen grains are compatible with the pistil as they germinated and pollen tubes grew through the stylar tissues and eventually entered the ovule for fertilization.
Sporophytic Self-incompatibility
Sporophytic self-incompatibility involves dominance and depends on the allelic composition of the plant that produced the pollen. Incompatibility is expressed at the surface of stigma.
In sporophytic self-incompatibility systems, the rate of pollen tube growth depends on the presence or absence of a dominant allele at the SI locus (will be called S locus in the following) carried by the pollen-producing plant. In the pollen, any S allele can exhibit dominance-that dominance is determined by the sporophyte, the plant that produces the pollen (thus, this system of self-incompatibility is termed “sporophytic”). Two important points need to be emphasized about sporophytic self-incompatibility.
The genotype of the pollen-producing plant determines self-incompatibility, not the allelic composition of the pollen itself. In other words, every male gamete has the same ability to fertilize a female as every other male gamete, irrespective of the pollen’s individual genotype.
There is no dominance in the female stigmatic tissue.
How does the genotype of the pollen parent transmit the influence of the dominant allele to the pollen?
Although we don’t yet understand this, we describe it as ‘imprinting.’ That is, the pollen “remembers” the genetic environment in which it developed and is conditioned to behave in accordance with that environment. Cell walls of pollen grains are consisted of at least two layers, the intine or inner layer and the exine or the outer layer. The exine is made up of a highly durable organic polymer, sporopollenin. The exine is believed to be derived from the somatic tissues of the pollen-producing parent and likely play a role in pollen-pistil interaction. This could explain why the compatibility response is determined by pollen-producing parent, instead of the pollen itself.
Here’s an example of sporophytic self-incompatibility. Assume the species is diploid and that there are four possible self-incompatibility alleles: S1, S2, S3, S4. Let S1 be a dominant allele. Let’s see what happens. (Color indicates the source of the allele, from the female or the male parent.)
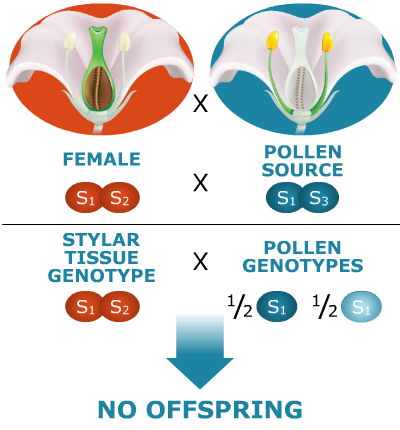
Why are no offspring produced?
The S1 allele in the plant producing the pollen is dominant. Hence, both the gamete types produced by that plant, S1 and S3, will behave as if they were both dominant alleles (S1). As a result, neither pollen type will be able to effect fertilization of this female—neither type of pollen tube will be able to penetrate the style because their growth will be impeded on the S1S2 stigma.
- In sporophytic systems, hindrance of pollen tube growth is localized on the surface of the stigma.
However, an exception is gametophytic self-incompatibility in several grass species such as rye and ryegrass, where pollen tube growth is inhibited on the surface of the stigma. - In gametophytic systems, growth is impeded in the style.
Pollen tube growth inhibition
A feature that distinguishes sporophytic from gametophytic self-incompatibility is the location of pollen tube growth inhibition.
When there are multiple S alleles in sporophytic systems, genetic segregation ratios become complex. The presence of a dominant allele in the pollen-producing plant conditions incompatibility if the female carries that same dominant allele. Assume a diploid species and dominance: S1 > S2 > S3 > S4.
Female Genotype | Male Genotype | Pollen Genotypes | Offspring | Explanation |
---|---|---|---|---|
![]() |
![]() |
![]() |
No | Matching alleles so incompatible |
![]() |
![]() |
![]() |
No | S2 is imprinted on S3 pollen grains. |
![]() |
![]() |
![]() |
Yes 1/4 S1S2: 1/4 S1S3: 1/4 S2S3: 1/4 S3S3 |
S2 is imprinted on S3 pollen grains. |
Self-incompatibility Systems
Many crops have self-incompatibility systems. Why might these systems have evolved? Self-incompatibility is common among naturally cross-pollinated species. Self-incompatibility prevents or limits self-fertilization and promotes out-crossing. Out-crossing maintains heterozygosity and heterogeneity in a population, which often improves plant vigor and productivity. In some species, homozygosity can severely reduce vigor, a phenomenon referred to as ‘inbreeding depression.’
Gametophytic Self-incompatibility | Sporophytic Self-incompatibility |
---|---|
Alsike clover | Sunflower (wild populations) |
Red clover | Buckwheat |
Tall fescue | Cacao |
Potato | Brassica species |
Rye (a two-loci system) | Cabbage |
Sugar beet (a four-loci system) | Broccoli |
Alfafa | Kale |
Tobacco | Brussel sprouts |
Not found in monocots! |
Techniques to Overcome Self-incompatibility
In order to self-pollinate or mate closely-related plants that are normally self-incompatible, plant breeders can employ various techniques that bypass self-incompatibility mechanisms. The technique used depends on the type of self-incompatibility.
Some systems have been developed or proposed for utilizing self-incompatibility genes to control pollination and produce F1 hybrid varieties.
Gametophytic System
Cross-pollination of vegetatively propagated, self-incompatible clones. Using this approach, seed can be obtained from species that are normally self-incompatible, and thus propagated vegetatively. An example is the production of a hybrid variety of bahiagrass, Tifhi.
Sporophytic System
Bud-pollination can be used in Brassicaceae for self-pollination and thus inbreeding, resulting in inbred lines or families homozygous for an allele at the self-incompatibility (SI) locus (only one SI locus is present in, e.g., cabbage). Hybrids can subsequently be produced by planting two such lines with different fixed SI alleles side by side. Any seed produced is expected to be hybrid seed, since self-pollination within parental lines is prevented by SI. The resulting hybrid will be heterozygous for the SI locus and thus self-incompatible. However, this is not critical since the economic product does not require pollination and is vegetative, e.g., cabbage and kale.
Pseudo-Compatibility
Produce inbreds in environments that promote pseudo-compatibility and their hybrids in environments that prevent self-fertilization. Sugar beet is normally self-incompatible. However, when grown at high elevations, plants are self-compatible.
Low Elevation: Sugar beet inbreds will behave as normal self-incompatible lines at low elevations — self-pollination is genetically eliminated. All seed produced at the lower elevation will be the result of out-crossing among inbred lines, and thus will be hybrid.
High Elevation: Self-fertilized seed can be obtained via elevation-induced pseudo-self compatibility. Sufficient quantities of selfed seed can be produced for subsequent hybrid generation.
Gametophytic System
- Pseudo-compatibility: Expose the plant(s) to lower or higher temperatures, elevated CO2 concentration, or electric shock to induce a pseudo-compatibility response.
- Sf alleles: Self-fertility (Sf) alleles, reported to be present in some species can be transferred into a population using conventional breeding methods. The presence of an Sf allele allows self-fertilization. These alleles can be present at self-incompatibility loci, but can also be present at distinct self-fertility loci.
Sporophytic System
- Removal of stigmatic surfaces: Mechanical (e.g., rupturing) or chemical removal of the stigma surface eliminates the inhibiting factors [believed to be specialized proteins or enzymes, (Barrett, 1998)] that inhibit pollen tube penetration of the style. With those factors absent, pollen tube growth can proceed normally and fertilization can be achieved.
- Bud pollination: Pollen is placed on an immature stigma before the inhibiting factor is formed.
An example of a gametophytic system is the production of a hybrid variety of bahiagrass, Tifhi.
Male Sterility
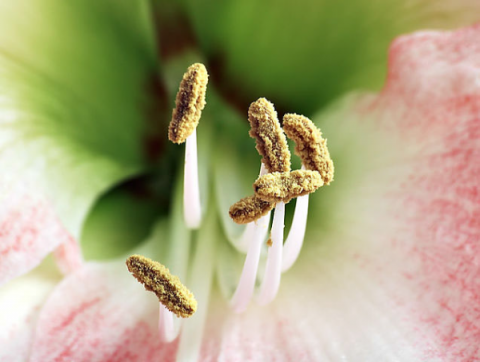
Male sterility is due to the failure of a plant to produce functional anthers or pollen; usually, its female gametes are normal. Thus, male sterility prevents self-pollination and can be used to ensure cross-pollination without emasculation. Male sterility can have genetic causes or be induced chemically.
Female sterility, the failure of a plant to produce functional ovaries or eggs, can also occur, but is of little use to plant breeders and will not be discussed.
Genetic-based Male sterility
Male-sterile gene expression may be complete or partial, and may vary with environment. Breeders desire complete expression that is stable regardless of environment. The extent of sterility is measured by the percentage of viable pollen produced or percentage of seed set. Male sterility is used by breeders to eliminate the necessity of emasculation to control pollination — male-sterile plants cannot self-pollinate.
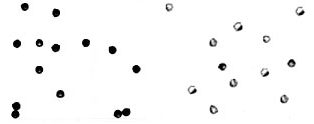
The percentage of viable pollen can be estimated using a microscope. Fresh pollen from a plant or group of plants is placed on a microscope slide and viewed at low magnification, 10X.
In the field, a hand-held magnifier is often adequate for scoring the percentage of viable pollen. Pollen grains can also be stained and viewed under a microscope in the lab.
Pollen viability can also be tested in bioassays.
Male-sterility is controlled by nuclear genes, the cytoplasm, or by genetic interaction between the cytoplasm and nucleus.
Controlled by the action of specific gene(s) in the nucleus. Usually, the recessive allele(s), designated ms, conditions inhibition of normal anthers or pollen development. Thus, the male sterility phenotype is expressed in plants homozygous for the ms allele.
- ms ms = male sterile (therefore, is functionally a female plant)
- Ms _ = male fertile (normal)
Maintenance of sterility genes in a population is challenging.
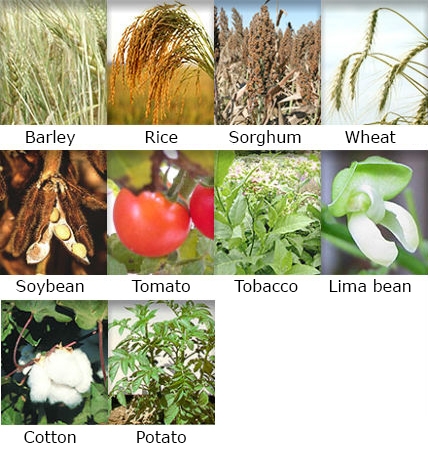
Numerous crops have genes causing male sterility in their gene pools.
There are two major uses of genetic male sterility:
- Eliminate hand emasculation in making crosses
- Increase natural cross-pollination in populations of self-pollinated crops
These uses necessitate the transfer of the ms allele into the population being worked with; then the ms allele is maintained in the population through selection for sterility in each subsequent generation.
Because genetic male sterility is controlled by a recessive gene(s), it is not possible to get a true-breeding or homozygous ms population. However, the recessive ms allele can be maintained at a high frequency in the population.
A male sterile line can be created and maintained by pollination of a line with an identical genotype except for the dominant allele for male fertility. To make the hybrid, male and female rows are planted in a specific pattern and selected plants are allowed to randomly mate with homozygous fertile plants.
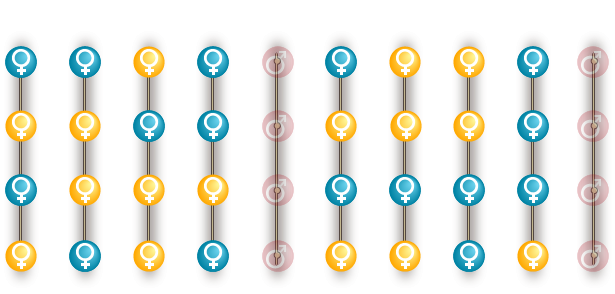
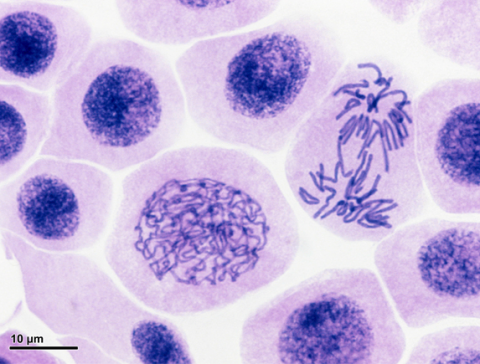
Cytoplasmic Male-sterility
The genetic composition of the cytoplasm determines male sterility. The genetic makeup of the cytoplasm results from genes located in mitochondria.
Cytoplasm is inherited entirely through the female line. The cytoplasm can be
- Normal (N) — normal development of anthers and pollen = male fertile; or
- Sterile (S or CMS) — anthers or pollen are non-functional = male sterile
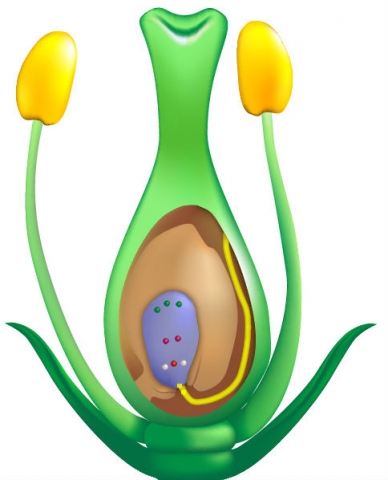
In all organisms, the cytoplasm contains genetic material in the mitochondria. Unlike the genetic material in the nucleus, however, cytoplasmic genetic material is not subject to recombination. Cytoplasm is inherited strictly through the female parent because the male parent does not contribute cytoplasm to the zygote. Recall that the zygote is formed when the sperm nucleus and the egg nucleus fuse; since the zygote’s nucleus is contained in the cytoplasm of the egg and the sperm has no cytoplasm, the zygote inherits its cytoplasm only from its female parent.
This fact is used by evolutionary biologists to trace family lines, avoiding the complexity resulting from genetic recombination and segregation that occur with nuclear genetic material.
In some systems, the expression of the male sterility phenotype depends on the interaction between cytoplasmic and nuclear genes. In these systems, a plant having sterile cytoplasm, but a nuclear dominant fertility-restorer gene (Rf _), will express a fertile phenotype. The particular combination of cytoplasm and nuclear genes determines the phenotype.
Cytoplasm Type | Nucleus Genotype | |
---|---|---|
Rf_ | rf rf | |
CMS | Male Fertile | Male Sterile |
N | Male Fertile | Male Fertile |
Plant breeders must pay close attention to both the cytoplasm type and nuclear genes of the parents used in crosses to generate and maintain cytoplasmic male sterility.
Sorghum Example
Several crops have cytoplasmic male sterility types available, including sunflower, millet, wheat, maize, and sorghum.
Let’s examine the maintenance and use of cytoplasmic male sterility in sorghum. This system involves interaction between the cytoplasm and a nuclear gene. There are two types of cytoplasm possible: S = sterile; N = normal. There are two possible alleles at the restorer locus in the nucleus, Rf (dominant) and rf (recessive). In this example, we’ll look at three types of inbreds.
Inbred Type | Cytoplasm Type | Nuclear Genotype † | Male Phenotype |
---|---|---|---|
A line | S | rf rf | Sterile |
B line | N | rf rf | Fertile (normal) |
R line | N, or | Rf Rf | Fertile (normal) |
S | Rf Rf | Fertile (normal) |
† Although we use rf and Rf in this example, sorghum breeders indicate the dominant and recessive nuclear fertility restorer alleles with different symbols: msc = rf and Msc = Rf.
How are these inbred (homozygous) lines maintained?
A line
Assume that the cultivar ‘Martin’ has two versions, one with normal cytoplasm and one that differs only in that it has a sterile type cytoplasm (‘Martin S’). The normal version is a B inbred type and Martin S is an A type. Mate the sterile version as the female with the normal, fertile version.
Notice that there is no blending or segregation of the cytoplasm. The F1 always has the cytoplasmic type of its female parent.
B line
B and R lines can be maintained simply by self-pollination. This is usually accomplished by growing each line in an isolated block and allowing the plants to intermate. Since the line is homozygous and fertile, the seed produced will also be homozygous and fertile.
R line
Like the B line, the R line can be maintained simply by self-pollination. This is usually accomplished by growing each line in an isolated block and allowing the plants to intermate. Since the line is homozygous and fertile, the seed produced will also be homozygous and fertile.
How is single-cross hybrid seed produced using cytoplasmic male sterility?
Cross a male sterile line (e.g., A line) by a fertile line (e.g., R line). ‘Caprock’ is an R line.
You’re planning to create a set of hybrids carrying various combinations of cytoplasms and fertility restorer alleles. A, B and R lines are available for generating the hybrids.For the cross shown below, predict the type of offspring, if any, will be produced.
Engineered Genetic Male Sterility
Different systems of male sterility and fertility restoration are being worked on by various companies. All of these involve molecular techniques (genetic engineering) and the development of transgenic plants.
One of the first to be utilized was the development of male sterile plant by transforming plant cells with a bacterial gene. This male sterility is dominant, since when the gene is present the plants are male sterile. The normal state for the plants is that the male sterile gene is not present and that gives normal, male fertility. As with the naturally occurring genetic male sterility we discussed earlier, it is impossible to maintain a population of completely male sterile plants as we can in cytoplasmic male sterility; so to identify the male sterile plants from the male fertile ones and to be able to eliminate male fertile plants, an herbicide-resistance gene has been linked to the male sterility gene. This resistance is also dominant, since when present it confers resistance and when absent (the normal state of the untransformed plants) plants are susceptible to the herbicide.
A plant is transformed to contain linked male sterility and herbicide resistance genes Ms R (The transformed plant will have only one homologue containing both the Ms and R genes, thus will neither be homozygous nor heterozygous, but rather what we call hemizygous for the two genes.)
We now must incorporate this linked pair into an elite inbred line by backcrossing. (The symbol “-” indicates that there is no allele for this gene present.)
The elite inbred will be developed and then maintained by crossing the male sterile plants with the normal inbred to give 50% sterile and 50% fertile plants.
Discussion for Further Thought
For the diploid, self-pollinated species tomato (Solanum lycopersicum), discuss from a genetic perspective the strengths and weaknesses of different hybridization systems that use either hand emasculation, male sterility, or genetic engineering, and come to a consensus, on which one you prefer.
Chemically-induced Genetic Male Sterility
In species that lack genetic male sterility or cytoplasmic male sterility, or in species in which these are difficult to work with, breeders can use chemicals to induce male sterility. These chemicals have at one time or another been called by the following names:
- Gametocides
- Pollen suppressants
- Hybridizing agents
These inhibit the production of viable pollen or prevent pollen shed, but do not damage the pistillate flower or interfere with seed development. There are both advantages and disadvantages to chemically-induced male sterility.
Advantages
- No need to develop and maintain cytoplasmic or genetic male sterility systems
- Applied to normally self-pollinated species, the chemicals prevent self-pollination and facilitate cross-pollination without the necessity of hand emasculation. This approach has been used in cotton, maize, sorghum, and various vegetable crops. It was also tested for hybrid wheat production.
Disadvantages
- Incomplete pollen sterility can occur, resulting in some selfing. Several factors can account for incomplete sterility.
- differential genotypic reactions to the chemical agents
- timing and dosage of application is critical and varies with genotype
- environmental effects on chemical and interaction with genotype
- long periods of flowering cause difficulties in maintaining optimum dosage in plants
- Does not provide a means of pollen transfer to produce hybrid seed, and so additional methods must still be employed.
Since wheat has perfect flowers and is normally self-pollinating, the production of hybrid varieties was impractical without the use of male gametocides. Gametocides were applied to the female plants prior to flowering to kill their pollen and prevent their self-pollination; the treatment was not applied to adjacent rows containing the intended male parent. Thus, seed produced by the female plants resulted from cross-pollination with the desired males. Although hybrid seed could be produced in this manner, the approach has been largely abandoned by most commercial seed companies—farmers did not buy the hybrid seed because the hybrids did not yield sufficiently better than conventional wheat to justify the additional seed costs.
Sex Inheritance
Description
The ultimate biological mechanism to prevent self-pollination, and thus inbreeding, is to have distinct male and female flowers on different plants. In such “dioecious” plant species, which are common in the plant kingdom, male and female plants occur usually at a 1:1 ratio. The decision on whether a plant becomes male or female is genetically determined, and can in some species be influenced by environmental factors. As a consequence, development of inbred lines can be accomplished either by crosses of male and female sister plants, or by employing environmental factors to induce plants with both sexes to allow self-pollination. In addition to dioecious and hermaphrodite plant species, there are various intermediate variants realized in plants (Table 4).
Plant Term | Definition of Plant Term |
---|---|
Sexually monomorphic | |
Hermaphrodite | Flowers have both male and female organs (Tomato, potato, sugarcane) |
Monoecious | Separate sex flowers on the same plant (Cassava, maize, banana) |
Gynomonoecious | Individuals have both female and hermaphrodite flowers |
Andromonoecious | Individuals have both male and hermaphrodite flowers |
Sexually polymorphic | |
Dioecious | Male and female plants (spinach, asparagus) |
Gynodioecious | Individual either female or hermaphrodite (papaya) |
Androdioecious | Individuals either male or hermaphrodite |
Genetics of Sex Inheritance
Distinct male and female flowers can be of practical importance, if present on the same plant. Best example is maize, where spatial separation of female and male flowers facilitates both elimination of male flowers to produce “female plants” for hybrid seed production, and self-pollination for inbred development. However, the majority of plants carry either flowers containing both male and female organs, or male and female flowers on distinct plants. In the latter case, pollination will be mediated by wind or insects. Female plants will set seed only if a male plant is located in the vicinity.
There are two genetic mechanisms of sex inheritance:
- Sex chromosomes — Like in humans, the sex of an individual is determined by specific chromosomes. In the case of humans, carriers of two X-chromosomes are females, whereas males have one copy each of the X- and Y-chromosomes. Since X- and Y-chromosomes differ cytologically, their pairing in meiosis might be incomplete.
- Autosomal inheritance — Genes affecting sex inheritance are located on “regular” chromosomes (autosomes), forming bivalents in meiosis. Depending on the plant species, sex determination can be due to one or a limited number of genes (mono-, or oligogenic inheritance).
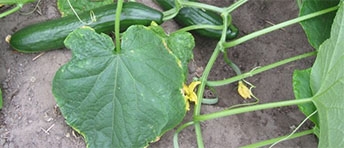
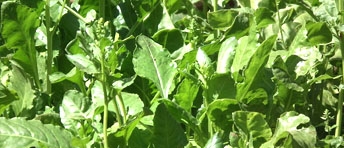
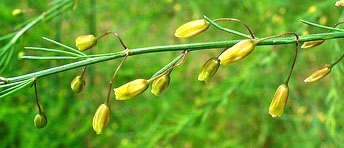
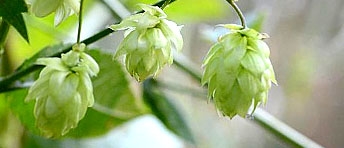
Application and Challenges
Application
Like self-incompatibility or male sterility, sex inheritance can be used for controlled crosses between any pair of genotypes in hybrid seed production schemes. One genotype would be used as female and seed parent, the other parent as pollen donor.
Challenges in using sex inheritance for hybrid seed production
As plants with only one sex cannot be sexually maintained, it is either required to use environmental or chemical factors to induce the other sex to allow self-pollination (if available), or to develop male and female sister lines for each hybrid parent, comparable to male sterile and maintainer lines when using male sterility. This is described in more detail in the following cucumber and asparagus examples.
Cucumber
Cucumber (Cucumis sativus) has a wide range of floral types. Staminate (male), pistillate (female), and hermaphrodite flowers can occur in different arrangements. Generally, embryonic flower buds possess both staminate and pistillate initials, and thus the potential to develop into any of the above-mentioned flower types. The cucumber phenotype with regard to floral types depends on autosomal genes and their interaction with environmental factors.
Sex inheritance is controlled by a minimum of three major loci:
- m+, m — controls the tendency to form hermaphrodite versus male or female flowers. mm homozygotes develop hermaphrodite flowers.
- F+, F — controls the female tendency, with F allele being dominant and favoring female flowers. This locus is subject to strong environmental influence. Among environmental factors, in particular photoperiodic conditions and temperature affect flower formation.
- a+, a — Homozygotes for a allele intensify male tendency. The effects of a are dependent on the allelic composition at the ‘F’ locus. Male tendency is only pronounced in ‘F+F+‘ homozygotes.
Although other loci such as ‘de’ and ‘cp’ have been shown to affect sex types in cucumber, the main sex types are determined by the above mentioned loci m, F, and a (Table 5).
Genotype, locus | |||
---|---|---|---|
Phenotype | m | F | a |
Androecious (male) | -/- | F/F | a/a |
Monoecious | m+/m+ | F/F | -/- |
Hermaphroditic | m/m | F/F | -/- |
Gynoecious (female) | m+/m+ | F/F | -/- |
CHEMICAL REGULATION OF SEX EXPRESSION
Phytohormones can be applied to alter flower phenotypes. Whereas auxin and ethylene promote female flowers, gibberellin promotes male flowers. Moreover, silver nitrate and silver thiosulfate induce male flowers even in strongly gynoecious genotypes. These treatments have become invaluable in cucumber breeding, as they allow efficient development of gynoecious inbreds to be used as female hybrid parents, and even the production of hybrids from crosses of two gynoecious lines.
Asparagus
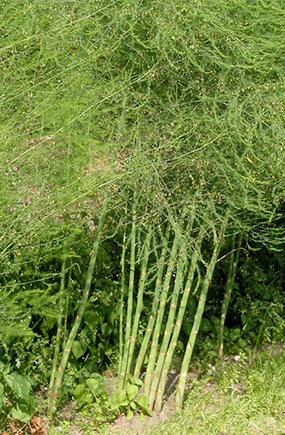
Sex inheritance in Asparagus officinalis, is similar to humans, based on sex chromosomes. XX chromosome carriers are female, XY carriers are male. Males and females occur at about equal frequencies in natural populations. Males have been shown to be more productive. For this reasons varieties with only male plants are preferred. At a low frequency and for unknown reasons from a genetic perspective, andromonoecious XY plants occur. These plants can be self pollinated, and will result in a 1:2:1 ratio with regard to the sex chromosomes in resulting offspring. One quarter of those will thus carry two copies of the “male” Y chromosome, and are thus called supermales. As Asparagus can be vegetatively propagated, those YY plants are potential variety candidates. Alternatively, they can be used as male parent in hybrid breeding schemes to be crossed with female XX genotypes, and result in 100% male XY offspring. In contrast, if regular XY males would be crossed to XX females, the offspring would segregate 1:1 into XY and XX plants, and XX plants would need to be eliminated by producers.
Controlling Hybridization
Knowledge of the breeding system of a crop species is essential to take advantage of the types of gene action that give the most useful cultivars. Self-incompatibility systems are important in many natural species for forcing outcrossing and thus maintaining vigor through heterozygosity. As we have seen, this system can be adapted to help produce F1 hybrid cultivars in domesticated species containing self-incompatibility loci. Similarly, sex inheritance can be employed in controlled F1 hybrid seed production schemes.
Male sterility is a rather unimportant method of enforcing outcrossing in natural plant species. It has, however, become such an important tool in the production of hybrid cultivars that it is utilized in many species of both cross- and self-pollinated crops.
References
Barrett, Spencer C. H. 1998. The evolution of mating strategies in flowering plants. Trends in Plant Science 3(9):335-341.
Charlesworth, D. 2002. Plant sex determination and sex chromosomes. Heredity 88:94-101.
Ellison, J.H. 1986. Asparagus breeding, Chapter 14, pp. 521-569, in M.J. Bassett (editor). Breeding Vegetable Crops. AVI Publ. Co., Westport, CT.
Goldberg, R.B., T.P. Beals, and P.M. Sanders. 1993. Anther development: basic principles and practical applications. Plant Cell 5:1217-1229.
Lower, R.L., and M.D. Edwards. 1986. Cucumber breeding, Chapter 5, pp. 173-207, in M.J. Bassett (editor). Breeding Vegetable Crops. AVI Publ. Co., Westport, CT.
Palmer, R. G., and M. L. H. Kaul. 1983. Genetics, cytology, and linkage studies of a desynaptic soybean mutant. J. Heredity 74:260-264.
Pierce, B. A. 2008. Genetics: A Conceptual Approach. 3rd edition. W.H. Freeman, New York.
A transformation useful on data collected of proportions or percentages. These data may be transformed by taking the inverse of the sine or arcsine of the number.