Chapter 1: Plant Breeders Rise to the Challenge of Feeding the World
Rita H. Mumm
Cultivars are the result of plant breeding, the science of applying genetic principles to improve plants for human use.
Plant breeding impacts the life of every individual because it involves the creation and manipulation of economically important traits in plants used for food, animal feed, fiber for clothing and wood products, fuel, and landscaping. Furthermore, plant breeding has been enormously successful! Average corn grain yields in the USA have increased from ~ 1.8 t/ha (~26.8 buA) in the 1930s when hybrids replaced open-pollinated varieties to ~11.7 t/ha (~174.6 buA) today. That is more than a 6-fold increase! The uniformity associated with hybrids is shown in Fig. 1.
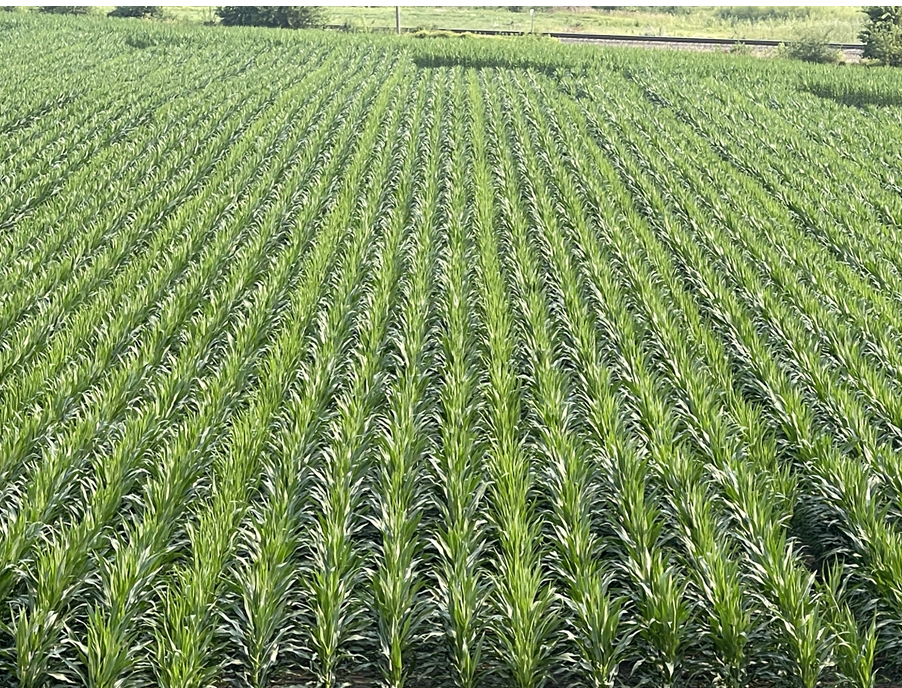
Global Grain Yield Increases
Globally, the average rates of grain yield increase per year for maize, rice, wheat, and soybean are 1.6%, 1.0%, 0.9%, and 1.3%, respectively. Observed global yields from 1961-2021 are shown in Fig. 2. Steady increases are evident with each crop, yet these rates of increase are not sufficient to meet the demand anticipated by 2050.
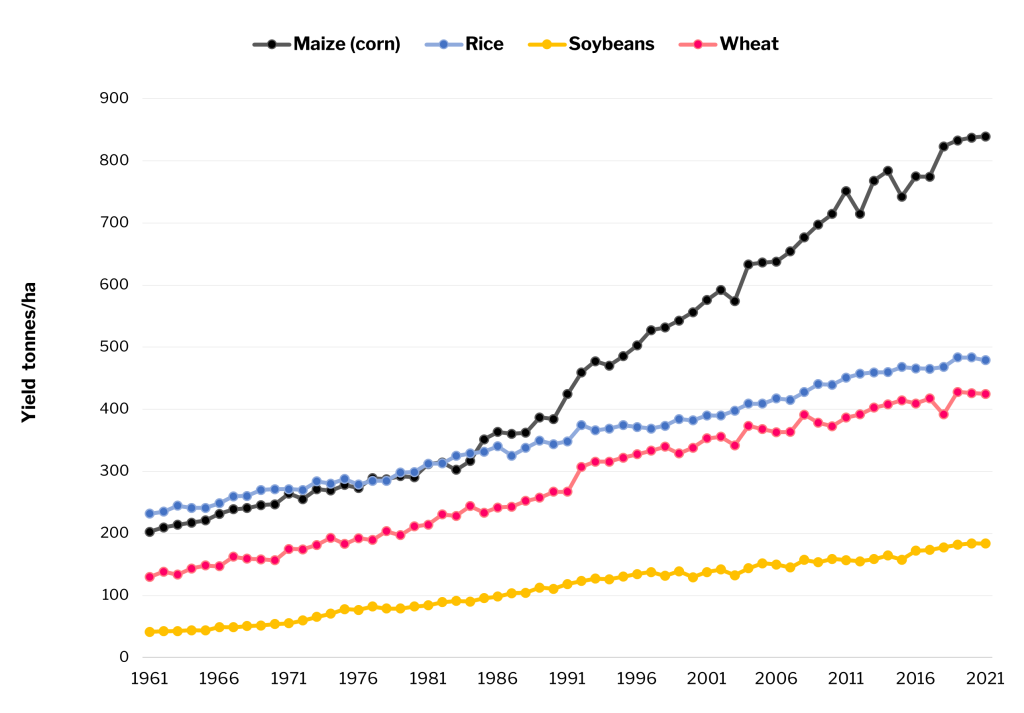
Grain Yield Increases to Meet Future Global Demand
A 2.4% per year rate of yield gain is needed across crops. The shaded area in Fig 2b shows the trend of the 2.4% yield improvement required each year to meet demand anticipated in 2050, without bringing additional land under cultivation, starting in the base year of 2021.
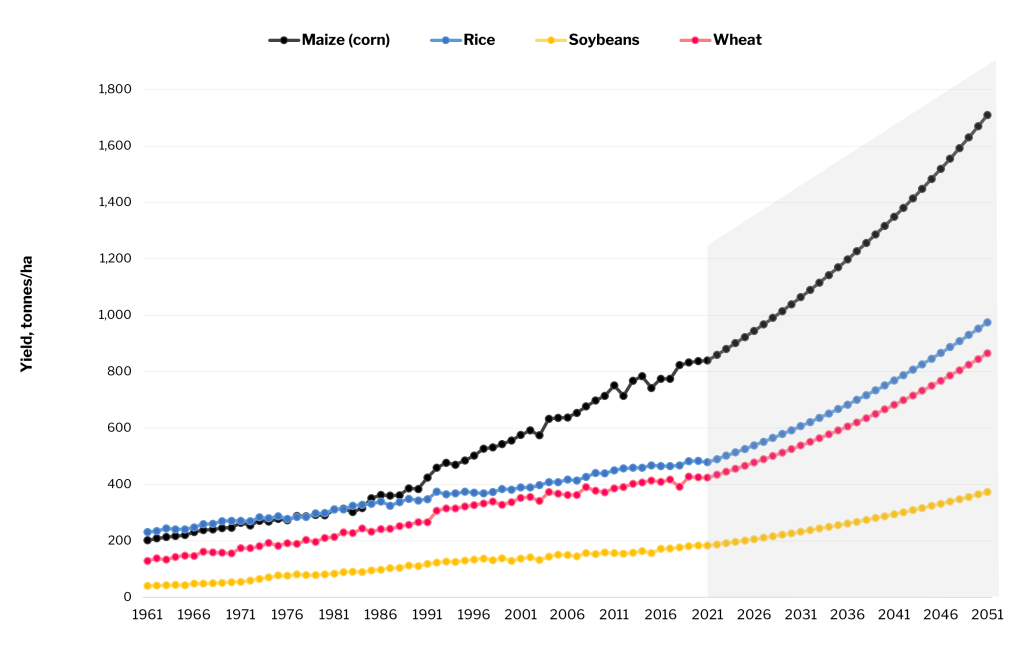
The Grand Challenge
The Grand Challenge that drives the development of improved cultivars is many-faceted:
- The world population is estimated at more than nine billion by 2050.
- Increased meat consumption in emerging economies as the standard of living increases.
- No appreciable change in available cropland globally and much of the available land is being degraded.
- Falling water tables globally.
- Climate change increases risk in crop production.
Crop yields must increase twofold by 2050 to meet the projected global demand for food and feed (relative to the base year 2008).
Maximizing Agricultural Production
What are some of the ways to achieve greater food production? How can agricultural productivity be improved? More land can be brought into cultivation (although this is not always feasible). Alternatively, more can be produced from each unit of land.
How is this accomplished?
- Improve the genetics of the seed.
- Better production practices to provide the sunlight, water, and soil nutrients plants need, and to mitigate stress factors.
Agricultural production can be maximized when the crop’s yield potential is manifested.
The Yield Gap
The yield gap considers the difference between:
- Yield potential, which is the yield productivity potential of an adapted cultivar when grown under favorable conditions without growth limitations from water, nutrients, pests, disease, and other stress factors, and
- Current realized yield, which is the actual yield on a specified spatial and temporal scale.
For more information on crop yield gaps, see Lobell, Cassman, & Field (2009).
A Glimpse of Yield Potential
The yield potential of a cultivar could also be considered in light of the biological productivity limit for its crop species, although plant breeders have not hit a permanent ceiling in any crop as yet!
U.S. farmer David Hula produced the highest corn grain yield on record, making him first place winner in the 2015 National Corn Growers Association contest. The 532.0271-bushel-per-acre (35.78 t/ha), certified yield on a 10-acre field was produced in Charles City, Virginia, under reduced tillage (no-till/strip-till) and irrigation with Pioneer hybrid P1197AM planted at a population density of 57,000 seeds per acre (54,500 plants per acre).
Genetic Challenges
Other challenges in plant breeding arise because most key traits of interest (e.g. yield) are polygenic. That is, typically many genes are involved in the expression of economically important traits.
- Each gene is thought to contribute a small effect.
- Genetic effects can be difficult to measure due to environmental noise.
- The expression of some genes is influenced by the environment.
- Genes of parents are randomly shuffled when a cross is made!
Undernutrition and Malnutrition
In addition to undernutrition caused by not having enough food, over one billion people in the world suffer from malnutrition (i.e. “hidden hunger”). Africa is particularly vulnerable. And, among the undernourished, children are especially hard-hit.
Status of Undernutrition and Malnutrition
Facts about undernutrition and malnutrition:
- 3.5 million maternal and child deaths could be prevented annually with improved nutrition.
- In developing countries, iron deficiency affects half of children under age 5, impairing growth, cognitive development, and immune function.
- Vitamin A deficiency affects at least 100 million children, limiting their growth, weakening their immunity, and in acute cases, leading to blindness.
- More than one-third of all African children suffer stunting (low height for weight, irreversible after age 2,) due to malnutrition and undernutrition.
- Stunting in early life is associated with lifetime debilitating neurological effects such as poor cognition and learning, low adult wages, lost productivity, and increased risk of chronic disease.
- Undernutrition, especially during the critical window from conception to 2 years of age, is associated with lower human capital.
- What is more, the devastating effects of malnutrition and undernutrition are across generations: a girl who was fed poorly as an infant is likely to have offspring with lower birth weight.
Crop improvement must be directed to producing better food as well as more food.
The Cycle of Cultivar Improvement
Generally, cultivar improvement involves the creation or assembly of useful genetic diversity and ways to exploit this variation to achieve targeted breeding goals.
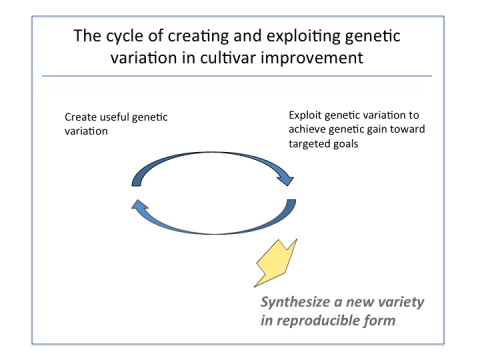
The general philosophy is simple: cross the “best” parents, produce progeny, and then identify and recover progeny that surpass the parents and demonstrate outstanding performance.
The superior progeny may become the basis of a new, improved cultivar; seed volumes (or plant propagules in the case of clonal crops) are produced for distribution.
Typically, this cycle is repeated multiple times to achieve a particular breeding target. Superior progeny may be used as parents in the next cycle, accumulating gains from selection.
Cultivar Improvement Challenges
Challenges include:
- Choosing the “best” parents.
- Identifying the truly superior progeny.
- Environmental noise that reduces heritability and makes it difficult to discern performance differences.
- Effective or efficient screens to measure performance for certain traits.
- A lack of knowledge about the metabolics and genetic architecture underlying the traits of interest.
Tools for Cultivar Development
Tools help!
For example, prediction can aid in choosing parents; transformation and gene editing may be used to create new useful genetic variation; doubled haploidy speeds development of homozygous progeny.
DNA-based technologies like molecular markers and sequence information enable genomics-assisted selection; analytics involves automated high-throughput analysis (think grain composition analysis); phenomics facilitates evaluation of performance in specialized testing environments (often focused on developing stress-tolerant cultivars).
Knowledge of Genetic Architecture Guides the Plant Breeder
With DNA-based information, knowledge related to genetic architecture and genome function then becomes a part of the cycle, leading to the creation of useful genetic variation and ways to exploit it in cultivar improvement (Fig. 4).
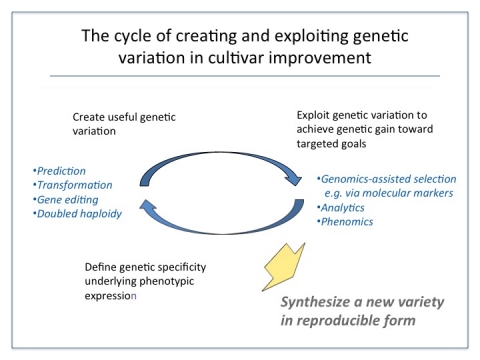
Increasing the Frequency of Favorable Alleles
Plant breeders (Fig. 5) are on a mission to increase the frequency of favorable alleles…
and to decrease the frequency of unfavorable alleles!
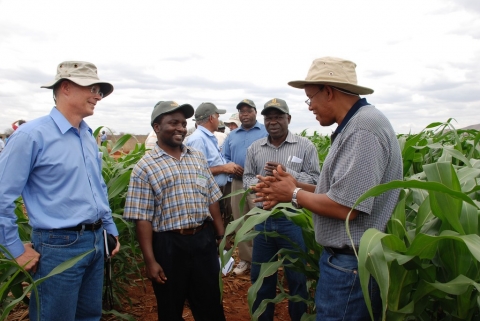
Fitness and Gene Frequency
In nature, there is natural selection for “fitness” contributing to the next generation (Fig. 6).
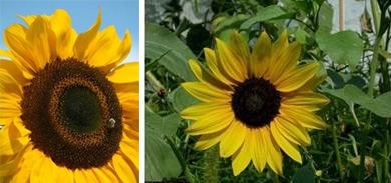
Selection and Selection Response
In deploying the cycle of creating and exploiting genetic variation to develop improved cultivars, the plant breeder implements basic genetic principles.
- Top-performing individuals from base population are selected as parents to produce the next generation, and that represents a complete cycle of selection (Fig. 7).
- Selection response ([latex]R[/latex]) depends on the total variation in the population, the bell-shaped curves in Fig. 7, the heritability (repeatability) of the trait ([latex]h^2[/latex]), and the selection pressure ([latex]S[/latex]) imposed. This relationship is represented in the Breeder’s Equation below.
[latex]R=h^2S[/latex]
where:
[latex]S=\mu_S - \mu[/latex]
where [latex]µ[/latex] is the mean of the base population and [latex]\mu_S[/latex] is the mean of the selected parents[latex]R=\mu_0 - \mu[/latex]
where [latex]µ_0[/latex] is the mean of offspring from selected parents
Rearranging the Breeder’s Equation gives heritability as:
[latex]h^2 = \dfrac{R}{S}[/latex]
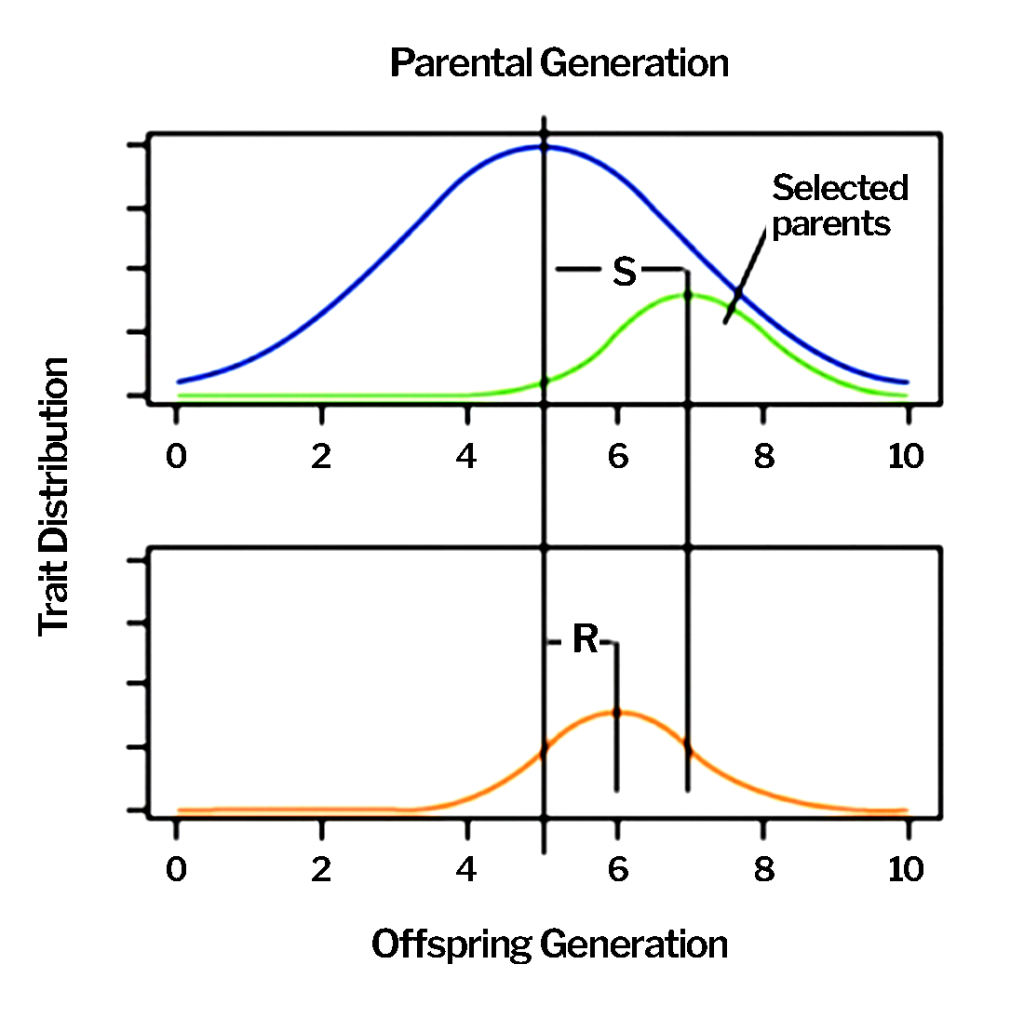
Rate of Genetic Gain
The rate of genetic gain depends on several factors (Fig. 8): heritability of the trait, the phenotypic variability in the base population, selection intensity, and the length of the breeding cycle.
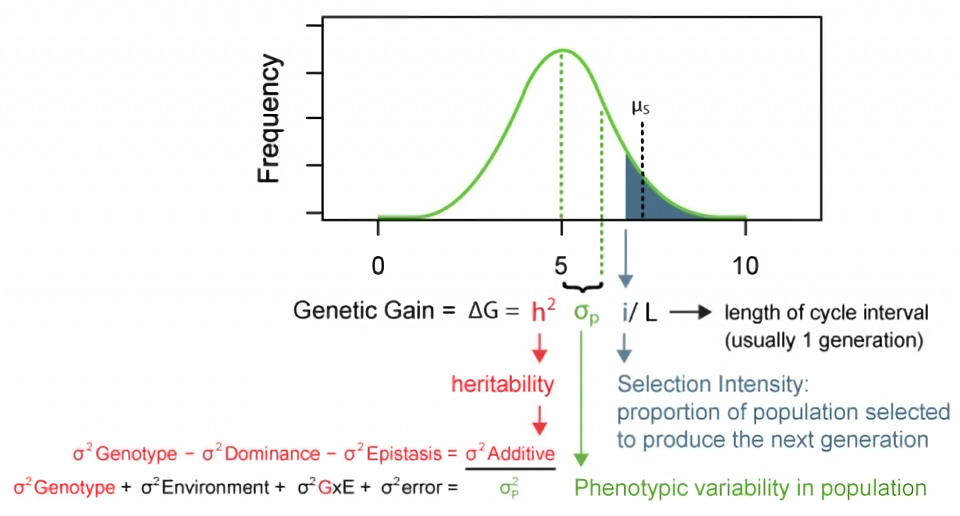
Implementing the Cycle
The plant breeder must rely on a systematic approach to effectively increase the frequency of favorable alleles for traits of interest and maximize the rate of genetic gain so that improved cultivars are available to farmers and producers on a timely basis.
Therefore, the breeder designs a process by which to implement the cycle.
The Cultivar Development Process
The process of cultivar development is:
- Established by crop.
- Enabled through tools, etc.
- Focuses on practical means to develop improved cultivars.
- Typically involves several steps spanning >10 years.
- Includes all steps from crossing parents to evaluating progeny to producing volumes of seed for distribution.
Given a finite amount of resources, the aim is to maximize genetic gain per unit of time and cost.
The process of developing improved cultivars involves 4 core functions that utilize different approaches to meet breeding objectives.
[Click on the plus sign + next to each of the core functions below to read about them.]
Note: Trait Integration is actually a special case of New Line Development and New Line Evaluation
The process pipeline and its core functions engage supporting groups and various facilities (Fig. 9).
[Click on the plus sign + next to each of the core functions below to read about them.]
Multidisciplinary Engagement
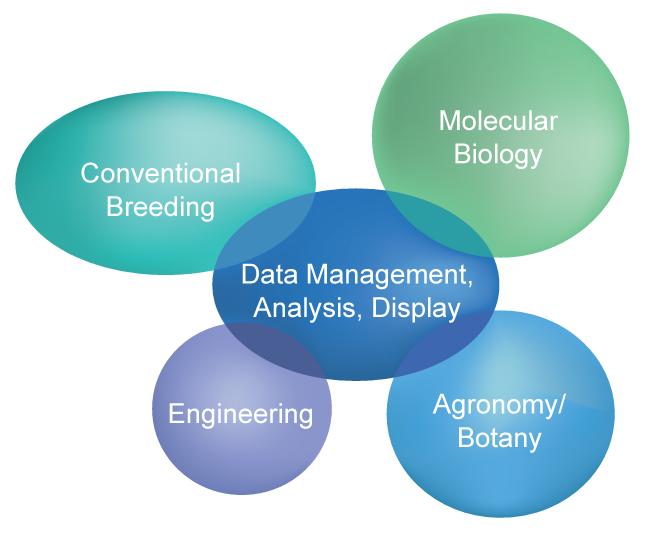
- Conventional Breeding
- Genetics: quantitative, population
- Plant breeding methods
- Selection theory
- Statistics & experimental design
- Knowledge of germplasm
- Phenotypic evaluation
- Molecular Biology
- Biochemistry
- Molecular genetic selection response (R) depends on the total variation in the population, the bell-shaped curves in Fig. 7, the heritability (repeatability) of the trait ([latex]h^2[/latex]), and the selection pressure (S) imposed. This relationship is represented in the Breeder’s Equation below.
- [latex]R=h^2S[/latex]
where:
[latex]S=\mu_S - \mu[/latex]
where [latex]µ[/latex] is the mean of the base population and [latex]\mu_S[/latex] is the mean of the selected parents
[latex]R=\mu_0 - \mu[/latex]
where [latex]µ_0[/latex] is the mean of offspring from selected parents
- [latex]R=h^2S[/latex]
- Genomics
- Transformation & tissue culture
- Sequencing
- Molecular marker technologies
- Gene cloning
- Data Management Analysis Display
- Bioinformatics
- Information technology
- Information management
- Computer programming
- Simulation & modeling
- Statistical and mathematical theory
- Engineering
- Profiling equipment
- Analytics e.g., grain composition
- Robotics
- Nanotechnology
- Agronomy/Botany
- Plant physiology
- Plant biology
- Soil science
- Pathology
- Entomology
Timely Delivery
To succeed in delivering improved cultivars to the marketplace on a timely basis, it is essential to design the process to:
- Align completely with stated product targets.
- Fully integrate all aspects.
Critical Decision Points
Critical decision points involve:
- Specifying your product target and your target market region.
- Choosing optimal parents to create breeding populations.
- Developing progeny with new gene combinations.
- Evaluating progeny to identify truly superior individuals.
- Selecting progeny to advance for further evaluation and to release as improved cultivars.
- Deploying tools and technologies for greater efficiency and effectiveness.
Designing the Process: Up-front Decisions
Before beginning any activities, the breeder is faced with some important upfront decisions:
- Specify the product target.
- Define the market region for the new cultivars.
- Identify base germplasm.
- Design the breeding strategy.
Determining the Product Target
Let’s talk about the product target… A breeder’s specific product targets will fall out of his/her organizational mission and research team goals. Whether working for a company or for a national program, the high-level organizational mission may look something like this:
You are part of a team effort!
Organizational Mission
In a seed company, the organizational mission is translated into an overall product goal, which may be a portfolio of products for a large region. For example:
And it may include more than one crop.
Individual Breeding Program Goals
An individual breeding program will have more specific goals, centering on a particular crop.
The breeder carefully and specifically describes what is desired as a net result of the breeding process.
For example:
Note that an individual program may have more than one product target.
What is a Product Target?
The product target describes the “What” and the “For Where.”
What characteristics does this product target imply?
- Maturity group 5 – 6 (i.e., early to medium).
- High seed yield.
- High yield stability (i.e. consistent performance across all types of environments).
- Minimal/no lodging (i.e., good standability at harvest).
- Minimal/no pod shattering ahead of harvest.
- RR1 transgenic event (which imparts tolerance to glyphosate herbicide).
Product Targets Indicate Specific Characteristics
Once the target characteristics are specified, the targeted levels of these characteristics and the way the characteristics will be measured must be specified. For example Table 1:
Characteristic | Measurement Standard | Threshold Level / Range |
High seed yield | Machine harvest; seed weight at 13% moisture basis, expressed per unit of land | 10% greater than Variety X |
High yield stability | Use regression analysis or GGE biplot analysis | Comparable to Variety X |
Lodging resistance | 1-5 scale; 1=plant erect, 5=prostrate | Score ≤2 |
Resistance to pod shattering | Oven dry method;
10 point scale measuring percentage affected; 0=none, 1=1-10%, 10=91-100% |
Score ≤1 |
Medium maturity | Maturity Group; day length and temperature required to initiate floral development; full range includes Group 000 to Group 9 | MG 5-7 |
RR1 event (Roundup Ready 1) | Integrate 40-3-2 | Pre-determined level of glyphosate tolerance |
The target levels for the target characteristics become the thresholds that will be used in selection.
For value-added traits like RR1, achieving the desired level of trait expression is typically a function of integrating the particular transgenic event through either backcross or forward breeding.
Product Targets Indicate a Specific Market Region
What market region is specified in this Product Target?
In this case, the market region is “dryland and irrigated areas of South Africa involving corn production.” Because the purpose of soybean in corn rotation is to fix nitrogen in the soil, certain bacteria must be present in the soil to facilitate this activity. Soybean works together with Rhizobia and other bacterial species to convert atmospheric nitrogen to a form readily usable by plants, presumably the corn crop in the following year. To ensure that nitrogen-fixing strains of bacteria are present, farmers may inoculate the soil. In this case, use of the inoculant “Rhizobia strain WB74” is specified as a production management practice.
These details speak to the population of environments, that is, the target market in terms of geography, production management system, season, maturity zone, altitude, etc.:
- Locations used as testing environments must be representative in terms of locations, planting dates, farmer practices, soil types, etc.
- Think of your testing environments as “samples” from the population of environments.
Measurements and Thresholds
Let’s look further…
The product target for an improved soybean variety for South Africa requires certain characteristics:
- Maturity group 5 – 6 (i.e., early to medium).
- High seed yield.
- High yield stability (i.e., consistent performance across all types of environments).
- Minimal/no lodging (i.e., good standability at harvest).
- Minimal/no pod-shattering ahead of harvest.
- RR1 transgenic event (the “Roundup Ready 1” event imparts tolerance to glyphosate herbicide).
And, with these requirements outlined, the breeder can designate the way the characteristics will be measured i.e., what “screens” will be used to evaluate performance for the trait. For example, to measure resistance to pod shattering, a protocol is needed for the “oven dry method” of evaluation.
The breeder can set the threshold levels for identifying and selecting superior progeny (Table 1).
Identifying Base Germplasm
The breeder also considers what germplasm to use as parents in creating progeny with useful, new gene combinations. Sources representing a high frequency of favorable alleles for the traits to be improved are needed.
Designing the Breeding Strategy
The breeder can even begin to consider breeding strategies to be used to assemble the suite of characteristics in one line.
Breeding strategies incorporate testing schemes and selection criteria, and also consider the following:
- mode of reproduction.
- selection intensity (i.e. how many individuals will be selected at each evaluation step) and order in which selection for particular traits is implemented.
- breeding methods.
- systems and facilities available.
- experimental designs for performance evaluation.
- predicted response to selection.
After all, the total package of characteristics is what will constitute the new, improved variety.
Types of Cultivars
In addition to the required characteristics and specific market, the product target indicates the type of cultivar to be developed.
There are several types of cultivars:
- A pure line variety is homozygous and homogeneous. It can be considered an inbred line. Example: soybean, pea.
- A hybrid is the result of crossing two genetically different lines. It is designed to exploit “heterosis”, or hybrid vigor, which may be expressed as increased yield and more robust plant health. Example: maize.
- An open-pollinated variety (OPV) is similar to a random mating population wherein cross-pollination occurs due to wind, insects, birds, and other natural mechanisms. Example: carrots.
- A synthetic is a population of cross-pollinated plants, typical of crop species that are self-incompatible for self-pollination. Example: alfalfa.
- A blend is a mixture of genotypes intended for genetic diversity to promote yield stability. The blend may comprise different types of disease resistance, slightly different maturities, or varying levels of winter hardiness. Example: wheat.
- Clonally propagated cultivars are genetically identical to a “mother plant” that is the result of cross-pollination. Often these species are polyploids. Example: potato.
Factors to Consider With Type of Cultivar
The reproductive system of the crop in question, its life cycle, and its ploidy level may dictate the types of cultivars that can be developed. For example:
- Obligate out-crossing species cannot produce pure line cultivars.
- Perennial crops include fruit tree species, many of which are produced from highly heterozygous parents; these are often reproduced and distributed as clones.
- Some seedless cultivars are the result of hybridizing parents of different ploidy numbers. Case and point: seedless watermelon results from crossing tetraploid with diploid watermelon to produce a triploid cultivar, which is used in production fields with a diploid pollinizer to produce sterile (seedless) fruit.
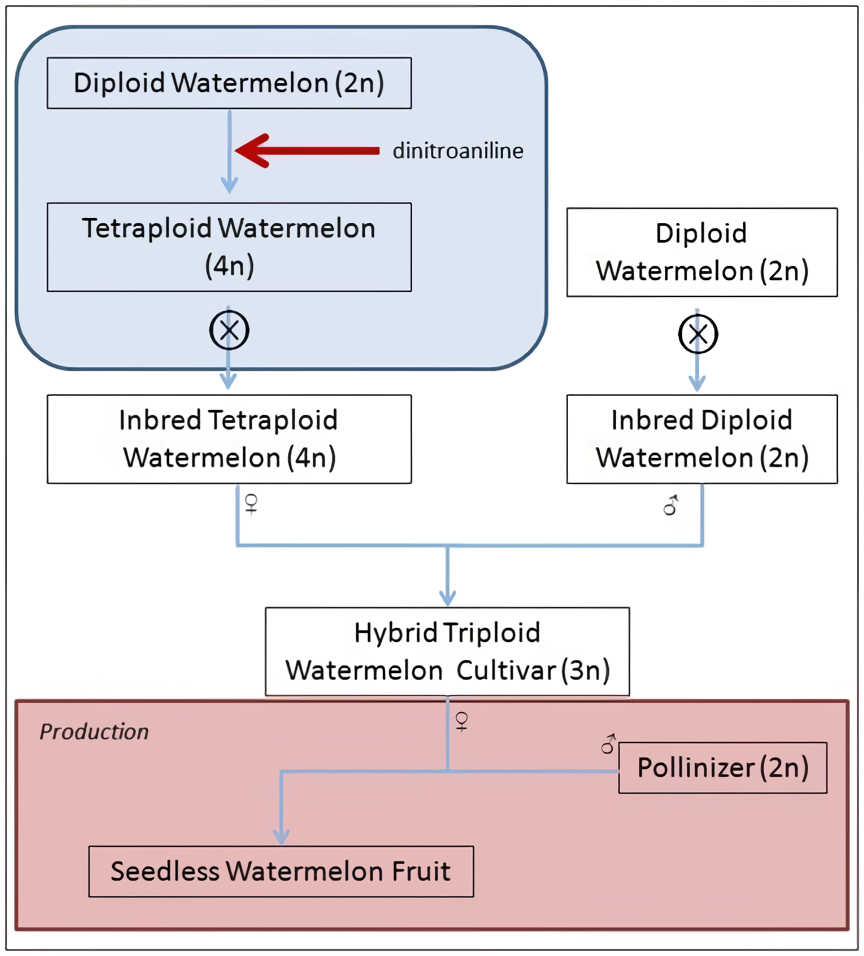
Hybrid Cultivars
Crops displaying heterosis may lend themselves to hybridization as a means to spike yields. Some crops that have transitioned from pure lines or OPVs to hybrids, or are in process, include maize, rice, sorghum, pearl millet, wheat, barley, sunflowers, cucumbers, tomatoes, melons, squash, and others. One essential requirement for hybrid cultivars is a dependable, cost-efficient means to control pollination in producing the hybrid seed (e.g. cytoplasmic male sterility, mechanical means to remove male flowers, environmentally- or chemically-induced male sterility, or a dioecious crop species).
In addition, farmers must be willing to purchase fresh seed each year (saved seed will not display the same levels of hybrid vigor).
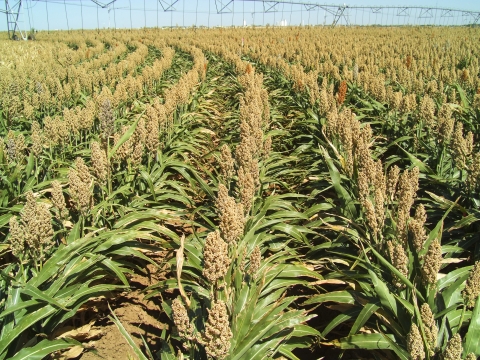
Process Efficiency
To recap…
The process of cultivar development becomes the mode and mechanism to implement the cycle of cultivar improvement effectively.
With the product target defined and the target market specified, the process must be designed to maximize the rate of genetic gain in order to accelerate the release of improved cultivars (Fig. 4).
[latex]\Delta G=\dfrac{ih^{2}\sigma_{p}}{L}[/latex]
Example: Commercial Soybean Improvement Program
Let’s look at an example of a commercial soybean breeding program. Note that this program serves a market region with one growing season (summer):
SEASON* | ACTIVITY |
Winter 0 | Make breeding crosses |
Summer 1 | Self or BC each population |
Winter 1a** | CONSISTENT use of SUBSCRIPTS
Grow 200 F2 or BC1 populations (i.e. S0 generation) that have been formed in previous years Advance the S0 plants to the S1 generation by a modified single-seed-descent method, retaining single pod (instead of a single seed) with 2-3 seeds; bulk by population |
Winter 1b | Plant S1 seed bulk;
Select 200-500 plants (~350 per population) and save selfed (i.e. S2) seed |
Summer 2 | Yield trials for 70,000 S2 families (across all populations) in unreplicated trials at 1-2 locations;
Select the best 5,000 based on yield performance; Save S3 seed from the trials |
Summer 3 | Yield trials for 5,000 S3 families at 3-5 locations;
Select the best 200 based on yield performance; Save S4 seed from the trials |
Summer 4 | Yield trials for 200 S4 families at 15-25 locations;
Select the best based on yield performance; code selected as ‘experimental’ lines; Save S5 seed from the trials |
Winter 4 | Increase seed of experimental lines |
Summer 5 | Yield trials of experimental lines at 20-40 locations
On-farm strip tests (i.e.150-300 m2 plots) at 20-100 locations |
Summer 6 | Yield trials of ‘advanced’ lines at 20-50 locations On-farm strip tests (i.e. 150-300 m2 plots) at 30-500 locations |
Fall 6 | Release 0-5 new varieties |
*Summer represents the main growing season, winter denotes off-season activities; the number after season indicates the year in the development pipeline
**Winter nurseries may be grown back-to-back in the same winter season
Notable Components
Components of the example soybean program:
- ≥7 years from the first cross to the commercial release of a new pure line variety.
- ~200 populations initiated per year.
- 500-1000 families per population.
- The number of new lines decreases as number of locations increases.
- 5+ years of yield testing before launch.
Course Roadmap
The remainder of this course will focus on how to design and implement the “process” of cultivar development so as to increase the likelihood of meeting your specified product target and identifying truly superior progeny among your breeding populations.
We will examine key decision points in the process pipeline and weigh the issues related to choices in:
- Choosing parents (to create breeding populations).
- Developing progeny (with new gene combinations).
- Evaluating progeny (to identify truly superior individuals).
- Selecting progeny (to advance for further evaluation and to release as improved cultivars).
- Deploying tools and technologies (for more efficiency and effectiveness) at various steps in the process pipeline.
In the remainder of this course,
- We will first walk through the “process” related to development of an improved pure line variety (the simplest example).
- We will then look at how the process might change if a hybrid cultivar is the target or if a clonally-propagated cultivar is the target.
- We will take a closer look at Trait Integration, which is a special case of New Line Development/New Line Evaluation where one parent serves only as the source of an important trait (e.g. preparing and utilizing sources of a value-added trait).
- We will explore ways to optimize the process pipeline to maximize gain from selection.
- Finally, we will examine aspects of product launch and producing volumes of seed for release and distribution of a new, improved cultivar.
References
Bernardo, R. 2010. Breeding for Quantitative Traits in Plants. 2nd edition. Stemma Press, Woodbury, MN.
Mumm, R.H. 2013. “A look at seed product development with genetically modified crops: Examples from maize.” J. Agricultural and Food Chemistry 61(35): 8254-8259. DOI: 10.1021/ jf400685y.
Ray, D.K., N.D. Mueller, P.C. West, J.A Foley. 2013. “Yield Trends Are Insufficient to Double Global Crop Production by 2050.” PLOS ONE 8(6): e66428. doi:10.1371/journal.pone.0066428
How to cite this chapter: Mumm, R.H. (2023). Chapter 1. Plant Breeders Rise to the Challenge of Feeding the World. In W. P. Suza, & K. R. Lamkey (Eds.), Cultivar Development. Iowa State University Digital Press.
A plant that has been produced through selective breeding and is maintained by propagation for release and cultivation.
Yield productivity potential of an adapted cultivar when grown under favorable conditions without growth limitations imposed by stress factors
A trait controlled by many genes
Hunger due to insufficient calorie intake
Deficiencies in the nutrients required for proper functioning of the human body, a condition which disproportionately affects children and women
The ratio of genetic variation to phenotypic variation
A term that refers to the metabolism of a living organism and the array of biochemical pathways and processes
The genetic alteration of a cell resulting from the direct uptake and incorporation of exogenous genetic material from its surroundings through the cell membrane(s).
A type of genetic engineering in which DNA is inserted, deleted, or replaced in the genome of a living organism using engineered nucleases, or "molecular scissors."
An emerging discipline dedicated to the measurement and study of phenotypic performance as the expression of the genome in response to a specific environmental.
Maturity group ranges from 000 (extreme north latitude) to 9 (near the equator) in soybean, with ranking based on photoperiod sensitivity and thus its flowering date (date that 50% of plants have begun to flower).
Tendency of the plant to lean or fall over, measured at harvest.
The propensity of a soybean pod to shatter during drying, releasing seeds before harvest time.
Source of a trait produced through transformation, which is defined by the unique DNA sequence that was inserted into the host genome as well as the precise point of insertion.
(1) Identical alleles are present at a locus on homologous chromosomes in a diploid or polyploid individual, such as AA in a diploid or AAAA in a tetraploid.
(2) Individual plants have identical alleles at each locus.
(1) Plants comprising the population are genetically identical.
(2) Population is comprised of genetically identical plants.
The number of sets of homologous chromosomes that make up the genome of a cell or organism. Each set is designated by n. For example, a tetraploid is 4n.
(1) Plant species having male and female reproductive organs in separate individuals.
Staminate and pistillate flowers are on different plants of the same species.
(2) Species with separate female and male flowering plants