Rivka Fidel
Learning Objectives
When you complete this chapter, you should be able to:
- Define ion exchange and CEC
- Draw a diagram showing how cation exchange works
- Explain the importance of cation exchange capacity (CEC)
Absorption and Adsorption
Sorption is the process by which soil retains nutrients and filters out contaminants. You can think of it as the soil “holding onto” chemicals. This is important because the more nutrients a soil can sorb, the more it can retain for plant use later, and the less fertilizer is wasted. Sorption also helps the soil retain contaminants, keeping them out of groundwater and nearby streams that are home to wildlife and/or used for drinking water.
As outlined previously, there are two types of sorption:
- Absorption = pores sucking up liquid that contains various dissolved compounds (solutes). The solutes then end up inside the pore, whether it’s between particles or inside a particle.
- Adsorption = solutes adhering or “sticking” to the outside of a particle
Because these terms sound similar, it’s easy to mix them up. Think of absorption as what a sponge does, absorbing liquids. Whereas, adsorption is more what sticky objects do, like a staticky ballon or microfiber cloth picking up dust.
Absorption vs Adsorption Analogy: Pie
Another way to think about absorption vs adsorption is to compare eating a pie with a pie to the face. Eating a pie is like absorption because the pie goes inside your mouth. Getting hit with a pie to the face is like adsorption because the pie filling sticks to the outside of your face. Below, Larry from the The Three Stooges demonstrates this analogy:
Absorption![]() |
Adsorption![]()
|
Absorption generally is proportional to water retention, which is discussed in the Soil Water chapter. However, adsorption is related to the surface chemistry of soil particles, especially colloids. Think – why would colloids and/or clay particles have greater effect than silt and sand particles?
Video Explaining Sorption Visually
Need a clearer picture of sorption? Check out this video:
Factors Affecting Adsorption
Adsorption to soil particles generally follows 2 rules:
- Like polarities attract like, meaning:
- Polar particles attract polar liquids or solutes
- Ex: Quartz and glass surfaces are polar, and attract water
- Ex: Smectite surfaces have polar groups that attract polar pesticides
- Non-polar solid particles attract non-polar liquids or solutes
- Ex: the waxy coating on a decomposing leaf is non-polar, and attracts liquid oils from elsewhere in the soil
- Polar particles attract polar liquids or solutes
- Opposite charges attract
- Negatively charged particle surfaces attract cations (positively charged ions)
- Positively charged particle surfaces attract anions (negatively charged ions)
This second rule of attracting opposite charges includes both very strong adsorption, such as heavy metals adhering almost irreversibly to colloids, and also weaker adsorption, that is reversible. This reversible adsorption is one of the most important soil processes: ion exchange.
Ion Exchange
Ion exchange is the rapid and reversible swapping of ions in the solution with oppositely charged sites on solid soil surfaces. It can happen at any solid-solution interface: where water meets a charged, solid surface. There are two kinds of ion exchange, named after the charge of the adsorbing ion:
- Cation exchange: the rapid and reversible swapping of cations in solution with cations on negatively charged surface sites
- Anion exchange: the rapid and reversible swapping of anions in solution with anions on positively charged surface sites
Not all ions are created equal. Some are exchangeable, whereas others bind too strongly and do not “unstick” or desorb from surfaces. Here are some of the most common exchangeable ions in soil:
- Exchangeable cations: H+, Al3+, Na+, K+, Ca2+, Mg2+, NH4+
- Exchangeable anions: NO3–, SO42–, Cl–
These ions play very important roles in soil. You may have noticed that most of these ions are plant nutrients: K+, Ca2+, Mg2+, NH4+, NO3–, and SO42-. Both Al3+ and H+ are acid cations that lower soil pH (more on pH and acid vs base cations in upcoming chapters). Additionally, Al3+ is toxic to plants. Na+ and Cl–, while non-toxic, are still important for affecting overall soil salt levels and the degree of soil clay flocculation and aggregation.
Both types of ion exchange are rapid because the aforementioned exchangeable ions do not bind directly to the oppositely charged surfaces, but rather keep a layer of water between them and the surface. You can learn more about this in the Advanced Ion Exchange Concepts chapter.
Note that this process is different from isomorphous substitution. Recall that isomorphous substitution occurs when ions from the solution replace ions inside a crystalline mineral like a phyllosilicate. Ion exchange, however, happens on the surface only.
Because 2:1 phyllosilicates are the most common and abundant in most soils, most soils have far more negative charge sites than positive charge sites. Consequently, we will focus on cation exchange in the remainder of this introductory chapter. You can read more on anion exchange in upcoming chapters.
How Cation Exchange Works
Picture a giant swarm of cations dissolved in water. They flow about with the water, and then suddenly encounter a negatively charged surface. They are attracted to the surface like magnets (although this isn’t actually magnetism). When they get closer, they encounter other cations already “stuck” to the surface. But some manage to “bump” some off, and replace them. That is cation exchange.

Cation Exchange Analogies
You can think of cation exchange as being like a game of musical chairs. Each chair represents a negative charge site, and each person represents a cation. The people walking around the chairs are like cations in solution, and people sitting are like adsorbed cations on the surface. Only one person can fit per chair, so only one cation can fit per site. (Technically, some charge sites can have a -2 charge, and hence either attract one +2 charged cation like Ca2+, or two +1 charged cations like K+. You can think of this as like a double-wide chair.)
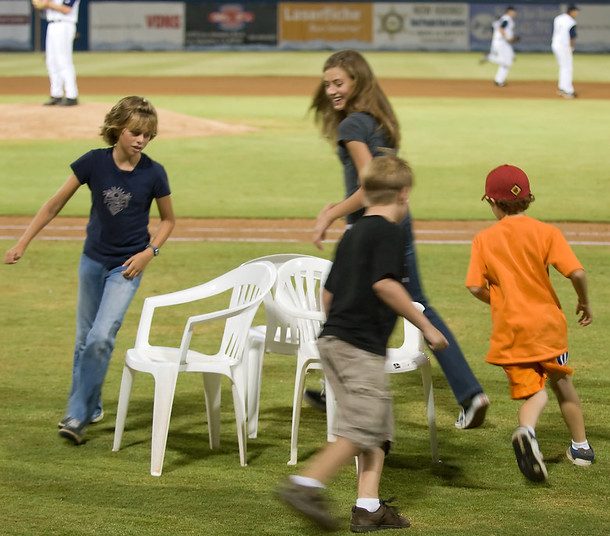
Another analogy is cats on laps. Most cats do not like to share a human lap with anyone. So, when a second cat approaches, the first cat leaves, and the cats swap just like cations. Here the lap is a negative charge site, and each cat is a cation.
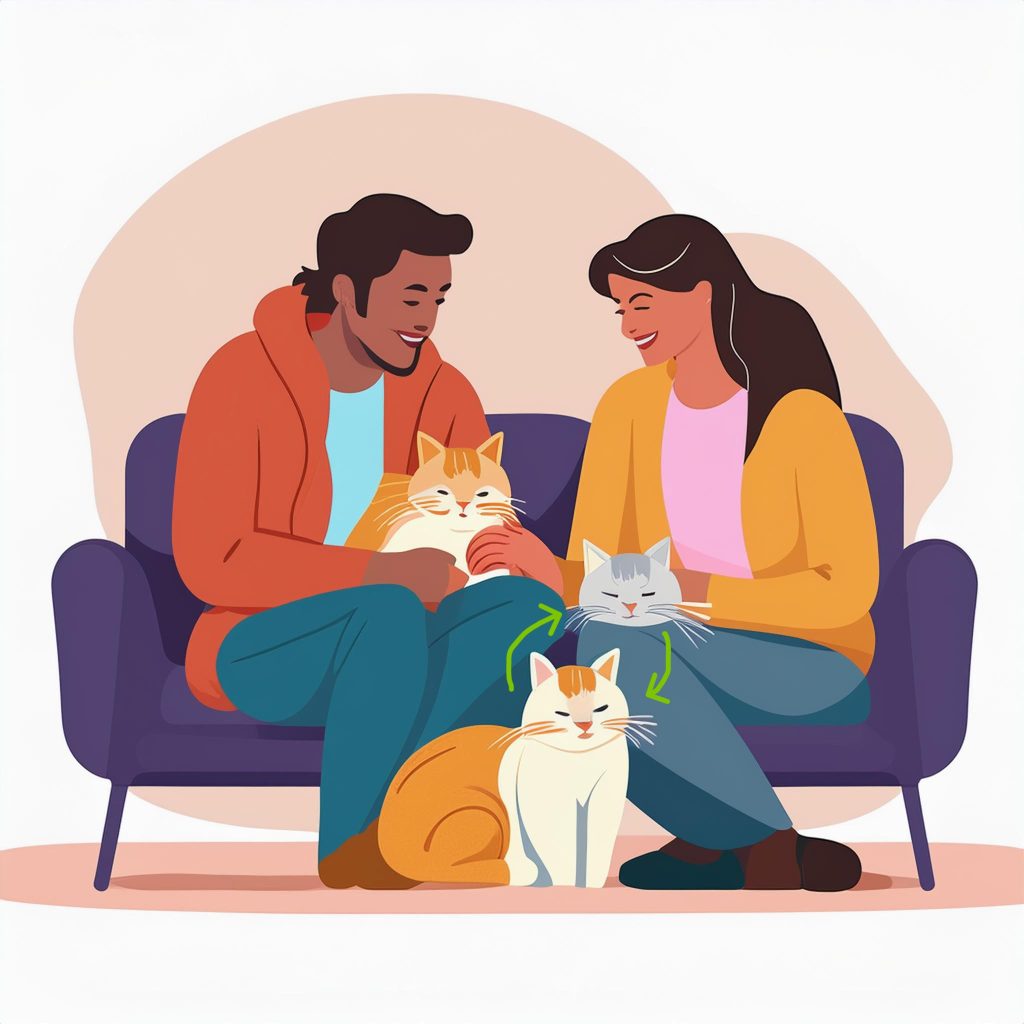
Like the other analogy, the cats-on-laps one works best when you consider +1 charge cations and -1 charge sites. But you can picture a +2 cation as a really big cat, and a -2 charge site as a large lap. One big cat, or two small cats, can fit on a large lap – just like one +2 cation or two +1 cations can adsorb to a -2 charge site.
Video Explaining Cation Exchange Visually
Need a clearer picture of cation exchange? Check out this video:
How Plants Use Cation Exchange to Get Nutrients
Adsorption occurring through cation exchange is very important for holding onto plant nutrients, because it keeps the exchangeable nutrient cations from washing away with rainfall. But how do the plants get the adsorbed nutrients back?
Plants are quite ingenious. They release their own cations, mostly H+, to swap with nutrients on soil surfaces (this relies on both pH effects and a phenomenon called “ratio law” discussed in upcoming chapters). Plant roots also produce CO2, which reacts with water to become carbonic acid (H2CO3), which then supplies even more H+ to exchange with nutrients when it dissociates. In other words, plants “bump” the nutrients back into solution with acid. Once in solution, the nutrients can then diffuse or move with the flow of water towards the plant roots.
So, the more cations the soil can “hold on” to via cation exchange, the more ultimately become available to plants, instead of washing away.
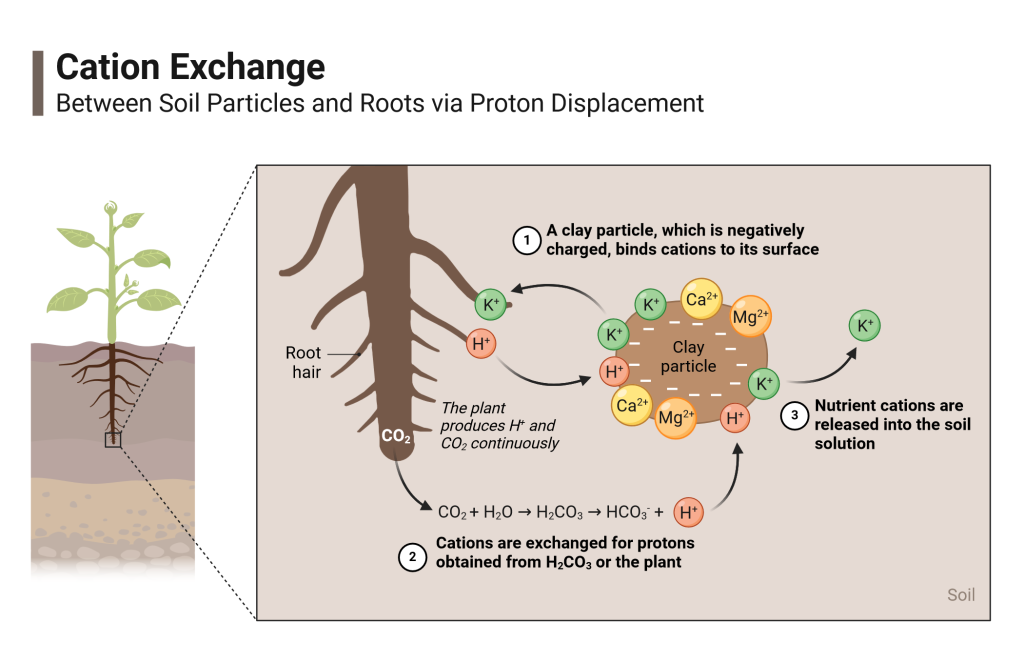
Cation Exchange Capacity (CEC)
The amount of cations a soil can retain through cation exchange is called cation exchange capacity (CEC). Because the amount of negative charge sites is equal to the amount of cations that can be exchanged, CEC is also equal to the concentration of charge sites per unit mass of soil. CEC is reported in units of centimoles of charge per kg of soil (cmolc/kg), which is equal to millimoles of charge (milliequivalents) per 100 g of soil (meq/100g).
Recall that different colloids had different concentrations of negative charge sites. The more concentrated the negative charge sites, the more cations a colloid can hold, the greater its CEC. In most soils, the majority of the negative charges come from phyllosilicates and other clay-sized mineral particles, as well as organic matter. The contributions of sand and silt are negligible. Thus, we can say CEC “comes from” clays and organic matter.
Diversity of Soil CECs Reflects Soil Diversity
Each soil has a different amount of organic matter, layer clays, and other minerals. The distribution of these important particles is like a “fingerprint” of the soil, in that it is unique – no two soils have the exact same chemical composition. Furthermore, each type of mineral itself can vary in its exact composition from soil to soil, as can the organic matter. For example, smectite in one soil may have undergone more isomorphic substitution than a smectite in another soil. Therefore, the CEC of a smectite in one soil can be different from the CEC of a smectite in another soil.
This is the case for each mineral, and for organic matter. Each one’s CEC can vary from soil to soil, as shown in the figure below.

Some of the minerals are more common in certain soil orders than other, based on degree of weathering and organic matter decomposition. As a result, some soil orders like Histosols have higher CECs than others. Compare the below table with the above figure, and think about the degree of weathering for each mineral as well as the organic matter in each soil order. Do you notice any patterns?
Soil Taxonomy order | CEC (cmolc/kg) |
---|---|
Ultisols | 3.5 |
Alfisols | 9 |
Spodosols | 9.3 |
Entisols | 11.6 |
Mollisols | 18.7 |
Vertisols | 35.6 |
Histosols | 128 |
There are 3 ways to determine CEC:
- Sum up the exchangeable cations, in cmolc/kg
- Take a weighted average of important colloids’ known CEC’s
- Cation displacement, where you flood the soil first with one cation, then another cation
See the next chapter for how to perform the calculations for each method.
Key Takeaways
- Ion exchange = the rapid and reversible swapping of ions in the solution with oppositely charged sites on solid soil surfaces. There are 2 types:
- Cation exchange: the rapid and reversible swapping of cations in solution with cations adsorbed onto negatively charged surface sites
- Anion exchange: the rapid and reversible swapping of anions in solution with anions adsorbed onto positively charged surface sites
- Exchangeable cations are available to plants because plants can use cation exchange to get nutrients.
- Plants produce H+ that swap with nutrient cations, forcing them into solution.
- The plants then take up the cations from the solution.
- CEC = cation exchange capacity = the total amount of cations a soil can retain in cmolc/kg