Soil water
Amber Anderson and Arturo Flores
- Describe where water is held in the soil
- Match water potentials to plant growth conditions
- Discuss factors involved in water movement into and throughout the soil
- Predict how water will move given soil conditions or properties
Overview
Water is intrinsically related to soil. It is a crucial resource for life, and an essential component of soil. Soil water is the content of water in an unsaturated system (also, the vadose zone), that is, the soil above the groundwater or the water table. Of particular importance is the nature of water and its interaction with the soil particles.
Water is held in the soil because of hydrogen bonding between water molecules and electronegatively charged soil particles (Gardiner and Miller, 1998). The water molecules consist of two hydrogen atoms and one oxygen. The way the atoms are arranged causes one side to be more negatively charged than the opposite, creating a polar molecule (Gardiner and Miller, 1998). The positively charged hydrogens (H+) are attracted to oxygens from other water molecules and/or to oxygens on the surface of the soil minerals and organic matter. Adhesion is the bonding between water molecules and solid particles. Cohesion is the ability of water to bond to other water molecules. The combination of these forces results in the capillary suction of a soil, which refers to the ability of water to move through the soil pores without the assistance of external forces, that is, in opposition to gravity.
Water flow is directly dependent on the soil texture and its pore distribution. First, water enters the soil by infiltrating through the soil surface, and then it percolates through the profile. For instance, water flow is faster with more granular and sandy soil than when the profile has a platy structure and heavier texture (clay). Several factors may be used to indirectly evaluate the hydraulic conductivity. However, for the purpose of understanding soil water content, the pore space is one of the main driving factors.
Soil porosity is classified according to the size of the individual pores. It is important to remember that water is held in between the soil particles due to the combination of adhesive and cohesive bonds. Capillary pores or micropores are the smallest of the system; mesopores are medium size; and macropores the biggest of all. Well-structured soils have an ideal pore distribution where micropores exist within the soil aggregates and larger pores in between aggregates (Schoonover and Crim, 2015).
Water potential
The water potential refers to the ability of water to do work and it could be an indirect measure of plant available water. It is measured in kilopascals or bars, and it is commonly expressed with negative value because water held in the soil has limited energy to freely move or react when compared to that of a saturated system (a pool for example). Water movement within the soil is limited by adsorption forces, solute or salt concentration, pressure, and gravity. The total water potential considers the effect of the following factors, and plants must overcome this tension to uptake water:
- Matric potential: water adsorbed to the surface of soil particles, or the one held in hygroscopic pores, has less potential energy. The matric potential is an indicator of the adsorption or retention force that the soil exerts over water molecules. In a saturated soil it generally has a value of zero. But, in unsaturated soil water is more tightly held and its value becomes more negative as the soil dryness increases. This means that the more negative the value is, or the higher its absolute value, water is less free and more energy is required to make it available. The matric potential is a measure of how hard water needs to be pulled to take it out of the soil system.
- Osmotic potential: the concentration of dissolved solutes or salts in the soil restricts the movement of water, especially if there is a membrane like a plant cell through which water must pass. It is always negative and its absolute value increases with higher loads of solutes. Plants need an extra load of energy to absorb water high in soluble salts.
- Pressure potential: pressurized water is more able to do work. Gasses and overhead water columns put pressure over underlying films of water. This causes water to flow faster and further than in a non-pressurized system. The pressure potential is zero when no additional pressure is applied, and it is positive when the system gets pressurized.
- Gravitational potential: refers to the effect of gravity on the energy of water. This is calculated based on the vertical position relative to a reference point. It has a positive value when the water is above the reference plane, and a negative value when it is below. For example, if a water reservoir is located 5 meters above-ground the potential energy will be positive, because it can freely flow downward due to gravity pull. The opposite occurs when water wants to be brought to a higher elevation. In this case, extra energy will be required to make it flow upward, and therefore, the gravitational potential will be negative.
Naturally, water is expected to move in favor of the water potential gradient, that is, from high water-potential (wet) regions to lower water-potential (dry) regions. This gradient indicates that water will flow following the difference in potential between two regions. Equilibrium exists when the water potential is equal throughout the system and there is no more water flowing across it.
In agriculture, water potential needs to be understood to guide irrigation management and achieve successful yields. The most common method to measure it is with a tensiometer (Ochsner, 2024). This is a device with a ceramic cup on one end that is connected to a water reservoir and a pressure gauge. When it is installed in the soil, water flows from the reservoir to the cup, and then out to the soil to equilibrate the moisture content between the cup and the soil. The pressure gauge measures the suction force applied by the soil to pull water out of the ceramic cup (referred to as tension). This occurs because as soil dries down there are more available charges that pull water stronger and closer to the soil’s particles, thus, increasing the matric potential. The tensiometer is not affected by solute concentration, it only measures matric potential. Still, for practical purposes, the matric potential is approximately equal to the total water potential (Miller and Gardiner, 1998), and this is a relatively inexpensive and simple device to improve water management decisions such as irrigation timing.
Soil water classification
For practical purposes soil water is classified according to its availability to plants. Soil holds water as films coating soil particles and in the pore space between them. Its availability is directly related to the soil water potential. Water becomes less available when the water potential is higher (more negative values, dry soil), and more available when the water potential is low (less negative, moist soil). In a saturated soil all the pores are filled with water. The water potential at this point is almost zero and it indicates that water flow is not restricted by the matric potential. This occurs after a significant precipitation event and is less dependent on the soil texture. However, the upper horizons do not remain saturated for long and the excess water freely drains away. Drainage takes time and while this happens, plants may used some of this moisture with minimum effort.
The gravitational water is that one that drains downward through macropores due to the force of gravity after soil gets saturated. This is temporarily available for plants, there is a low absolute matric potential affecting, and it takes between 24 to 48 hours for it to become negligible. After free drainage the soil remains wet but not saturated, and air occupies pore space, especially that of the macropores. At this point, soil is said to have reached field capacity.
Field capacity is defined as the maximum amount of water that a soil can hold after gravitational drainage (Gardiner and Miller, 1998). Water is held with a tension of -0.1 to -0.3 bar (-10 to -30 kPa), and plants can easily pull it out of the system. The plant available water is the moisture content immediately available for plant uptake. Its upper limit is set by the field capacity and the lower limit by the permanent wilting point. Gravitational water is insignificant at field capacity; however, some can still drain but at a much slower rate. Evapotranspiration increases the absolute water potential by reducing the moisture content of the soil and making the remaining less available for plants. The permanent wilting point is reached when water is held with a tension that plants cannot overcome, which is about -15 bar (-1,500 kPa). Plants start to wilt and they may not be able to recover their turgor. A considerable amount of water remains in the soil at this point but is not available for plants. The reason for this is that water is held in the soil’s micropores, and it is held with a tension that exceeds the plant’s ability to remove it. This is called hygroscopic coefficient (also hygroscopic water within capillary pores) and water is held with a tension close to -31 bar (-3,100 kPa).
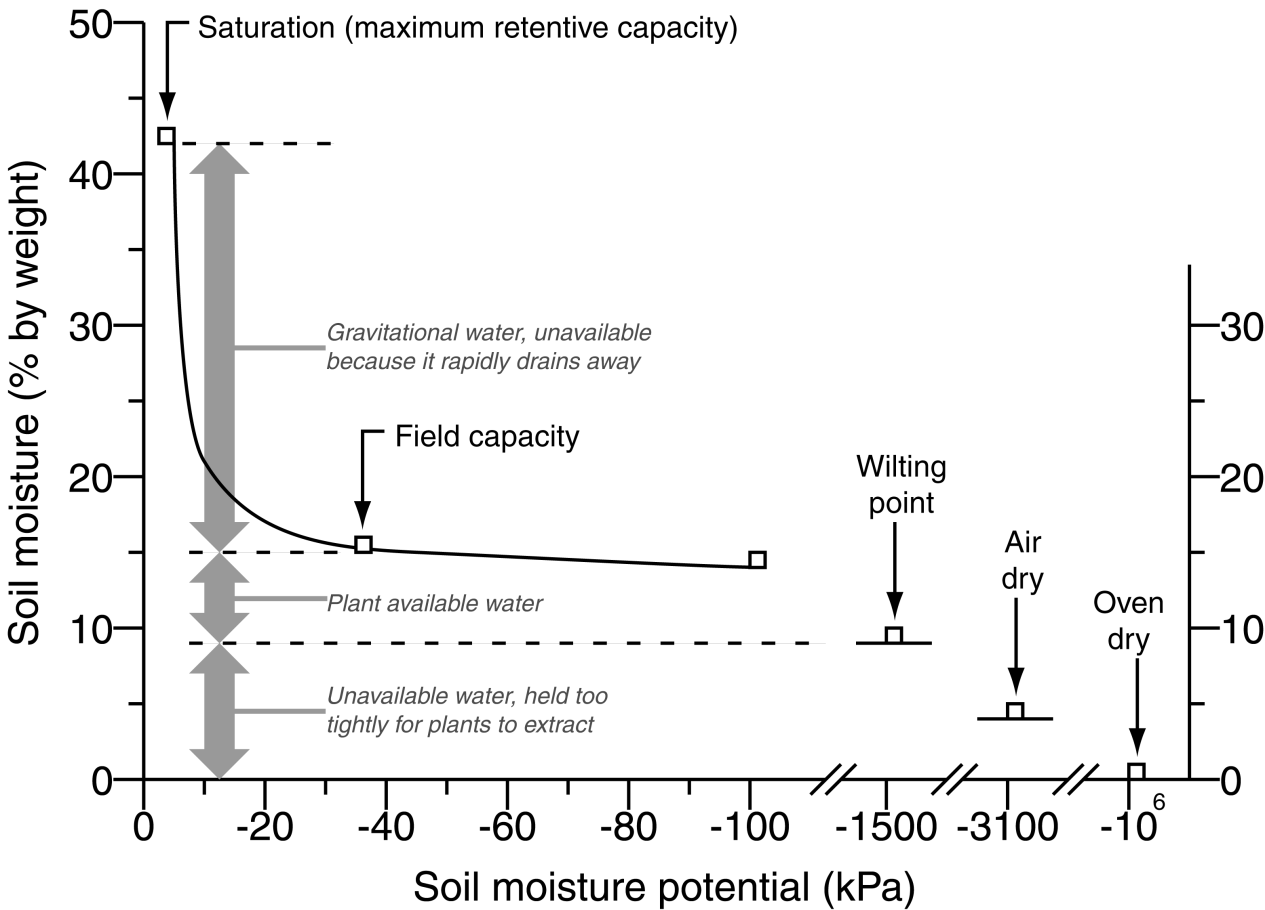
In practice, plant available water directly depends on the soils texture. Colloidal soil particles (clay and humus) are able to hold more water than sandy soils. In agriculture permanent wilting point should be avoided at all costs to prevent yield reduction. Therefore, soil is allowed to lose only a tolerable percentage of its plant available water before irrigation occurs. This is called management allowable depletion. Unlike permanent wilting point that is a set as a function of retention forces, the allowable depletion threshold is set as a function of the crop’s tolerance to drought. It is calculated by subtracting a percentage of the plant available water from the field capacity, in terms of tension or volumetric water content. Soil moisture is monitored with soil sensors, like tensiometers, that provide users with an approximation to soil water content. This can be later related to volume of water per volume of bulk soil and help monitor irrigation practices.
Soil water content
Air- and oven-dried soils are two concepts that may be found in literature. These refer to the process applied to reduce the moisture from a soil sample. The air-dry method consists of exposing the sample to ambient air or to room temperature (20 – 25 °C) to let moisture evaporate. This is similar to what will occur naturally in a soil, which is a function of evapotranspiration and gravitational drainage. A thin film of water still remains in the air-dry soil, but it is not readily available for plant uptake. This indicates the water held with a tension of -31 bar (-3,100 kPa) and may be associated with the permanent wilting point. The oven-dry process puts soil in an oven at a temperature between 100 – 110 °C until weight remains constant. The extra energy applied to evaporate moisture from the sample makes the remaining water content be held to a tension up to -100 bar (-10,000 kPa). This process is applied to soil samples when further laboratory analysis is desired, such as particle density or nutrient extraction. Extra temperature applied beyond the recommended ones may risk the composition of the sample, altering the soil’s mineralogy and organic matter content. This could be a problem if chemical analysis are performed afterwards. Both of these methods are disruptive procedures, do not allow repeatability, and require time and equipment, limiting its applicability for practical purposes (e.g., crop production).
Another way of communicating soil water content is on a mass or volume basis. Direct measurements include gravimetric (θg) and volumetric water content (θv). The gravimetric method measures the mass of water in a given mass of oven-dry soil (g g-1). The mass of water is the difference in weight between the soil sample before and after drying it in an oven. The volumetric water instead indicates the volume of water contained in a given volume of dry soil. This is calculated by multiplying the gravimetric water content by the bulk density of the soil, assuming that the water density equals 1 g cm-3. It can be expressed as unitless decimals (cm3 cm-3), or as a percentage if multiplied by 100. Additionally, the thickness or depth of water in a soil layer is given as the soil water storage. This one is calculated by multiplying the volumetric water content by the depth of the soil layer (e.g., rooting depth).
In agriculture a popular instrument to measure the volumetric water is called TDR (Time Domain Reflectometry). This device sends an electric signal through some metal probes of known length that are inserted into the soil, and measures the speed at which they travel. The time it takes the signal to go back and forth across the probe is proportional to the dielectrical constant of the soil, in drier soil it takes longer than under wetter conditions. The process is more complex than what is explained here, but the advantage of these devices over soil-drying methods is that they allow for instantly repeating measurements at the same location without disturbing the surface. In the golf industry TDRs include also electrical conductivity and temperature sensors that increase the level of detail for managers to take more accurate decisions.
Soil water demonstration
Watch this overview video at: https://youtu.be/m4iHBy5PNY4
References
Miller, R.W. & Gardiner, D.T. 1998. Soils in our environment. Prentice Hall. 8th edition. ISBN 0136108822.
Ochsner, T.E. 2024. Rain or shine An introduction to soil physical properties and processes. Department of Plant and Soil Sciences, Oklahoma State University.
Schoonover, J.E. and Crim, J.F. (2015), An Introduction to Soil Concepts and the Role of Soils in Watershed Management. Journal of Contemporary Water Research & Education, 154: 21-47. https://doi.org/10.1111/j.1936-704X.2015.03186.x
- Water is held in the soil as a result of cohesion and adhesion forces.
- The soil water potential indicates the strength or energy required to remove water from the soil, and it is an indirect measurement of water availability.
- Plant available water is the difference between field capacity and permanent wilting point, and it is readily available for plant uptake.
- Water content may be communicated either as gravimetric or volumetric water content.