Soil geography
Bradley Miller and Arturo Flores
- Define soil geography as a tool of soil science.
- Understand the importance of spatial variability of soil.
- Discuss the interest of soil science to create soil maps.
Keywords: soil geography, spatial variability, soil map, dynamic properties.
Soil geography
If not all soil is the same and different soils have different capabilities, then it becomes important to know where these different soils exist. So, to match land use and management planning to the capability of soil, we need to understand the spatial distribution of soils. In addition, the soil is changing. Some soil properties change faster than others and they are dynamic both in space and time.
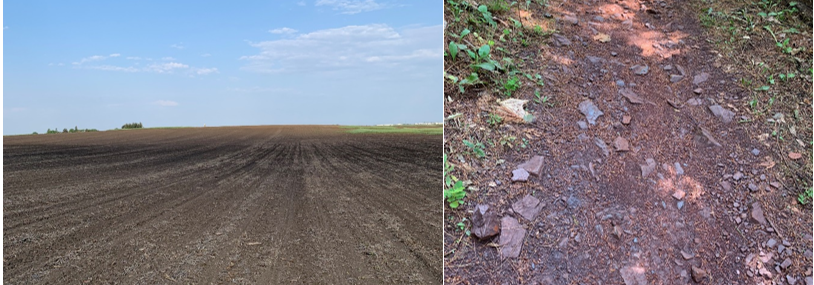
The spatiotemporal variability of soil is the result of different forming factors, weathering rates, time of development, and human intervention. It is known that soil not only varies vertically (soil profile), but laterally (across the landscape). Variability is easily observed from one place to another (macroscopic variability), or it can exist at a microscopic level. However, despite the level of detail at which soil is being evaluated, heterogeneity exists and is critical for land management. Therefore, it is possible for geography to study soil as a geographic unit.
Soil geography applies geographic principles into the spatial study of soil heterogeneity. Soil properties or characteristics are a response to their location. Soil forming processes occur differently across the landscape. The location of a point the geographic space is determinant for weathering intensity. The fundamental concept of ‘catena’ was explained by Geoffrey Milne, who defined it as ‘the regular repetition of soil profiles in association with certain topography.’ This concept captures the impact slope has on hydrology, and how the last serves as a critical factor for soil dynamics.
The most influential factor in weathering and sediment translocation is slope gradient. This is the inclination or degree at which a slope gains or loses elevation compared to the horizontal level. It can be measured in degrees (45°) or in percentage (100 %), meaning that a slope of 100 % has an inclination of 45 ° and gains one unit of elevation for every horizontal unit. Steeper slopes increase water kinetic energy and its erosive potential, whilst gentle slopes or almost level ground decrease the speed of water fluxes and increase material accumulation.
Convex slopes tend to be easily eroded from surface particles that accumulate more in concave regions. Higher deposition may be correlated with high enrichment areas as sediments carry nutrients along. However, they can also be problematic as water tends to accumulate more and optimal rooting conditions can be limited. Slope orientation has a regulating effect on soil properties. Regions facing to the south (in the northern hemisphere) and to the north (in the southern hemisphere) are exposed longer to solar radiation. This results in higher soil temperatures that increase biological activity, production of biomass, and perhaps reduce the time soil remains frozen after freezing winters. Wind also impacts soil development, and the east-west orientation of the slope is therefore critical in wind erosion. Slopes facing directly to the wind current are more prone to suffer from erosion than slopes on the other side of the hill. Assuming that wind is flowing west to east, a slope facing west will be slowly depleted from fine surface particles and will end up with higher accumulation of coarser materials, whether opposing slope (facing east) might end up less disrupted and with more fine sediments.
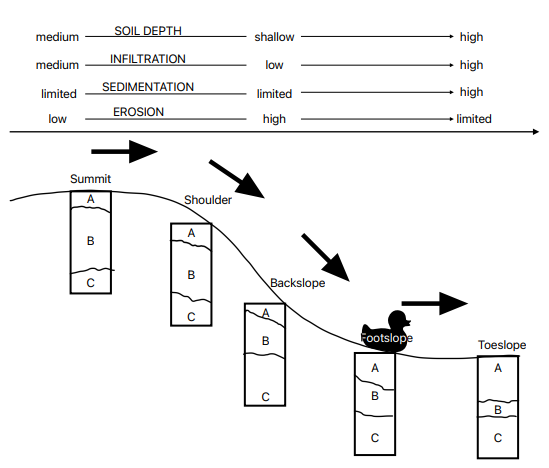
The position on a slope will also determine the speed at which water can erode surface materials, the infiltration rates, and the accumulation of sediments. From top to bottom, hills can be divided into summit, shoulder, back slope, foot slope, and toe slope. The first two correspond to less slope steepness (inclination) and have higher infiltration rates. Where slope starts to increase, water potential increases and with it, runoff. The back slope is the steepest section of a slope, and this causes finer particles to be carried down through water fluxes (surface water erosion). This region does not allow much time to infiltrate and has higher translocation of finer materials. Therefore, back slopes have higher content of sand or gravel and less developed soil horizons. As the slope starts to settle, the foot slope and toe slope are located at the bottom of it. Here steepness is reduced and water potential decreases as its speed also is reduced. This causes the sediments to settle and increase the thickness of the surface materials, which can include silts, clays, and nutrients carried along. The previous image demonstrates the sections of a slope and what processes are more likely to occur in each one of them.
Soil maps
We use soil maps for many things. The original purpose of soil maps was cadastral, which means land valuation for the purposes of taxation. The idea is that owners of land with more productive soil will have higher yields, higher income, and thus can afford to pay a higher portion of taxes. This use of soil maps is still common today for agricultural land. Another fundamental purpose for soil maps is interpretations for land use capability. Some of the earliest soil surveys were part of geologic reports and were essentially inventories of natural resources. This was especially common for colonies and other lands newly set up for settlement. In the early history of the USA, European settlers were setting up new farms and the soil survey maps provided guidance for which crops were best suited for that land. These reports were typically organized and paid for by county and state governments.

Another common type of soil map that serves a different purpose than soil survey maps are soil fertility maps. These soil maps focus on soil properties that change quickly and support decisions that are important to the annual economics of field management. To differentiate between long-term and short-term soil properties, we categorize them as static and dynamic soil properties. Soil survey maps focus on static soil properties because they are more reflective of the natural capability of a soil. Also, given that soil survey maps have taken a long time to create for the large extent they cover, they focus on soil properties that won’t make the maps out of date only a few years after they are created. In support of the cadastral purpose of soil survey maps, focusing on the natural capability of soils avoids the variability in management. In other words, the taxation rate is based on standard management practices, not if the farmer is especially good or poor at managing their fields.
The dynamic nature of soil fertility properties makes them more challenging to predict. While the factors of soil formation still apply, the relationship with those factors changes over time. For example, the application of a nitrogen fertilizer may level soil nitrate concentrations across a whole field. As time goes by, plants are consuming some of that nitrate and other biological processes are converting it to nitrous oxide gas (denitrification). While the plants may be taking up nitrate at spatially consistent rates, denitrification occurs at different rates depending on spatially variable factors such as soil water content. In addition, water moving through the soil leaches nitrate downward. Sometimes that leaching takes the nitrate straight down and sometimes that water transport is more lateral, causing the nitrate concentrations to decrease in the upper elevations and increase in the lower elevations. At any particular point in time, we could measure soil nitrate concentrations by soil sampling and identify patterns with respect to landscape position. However, as the nitrate migrates down the slopes the relationship with landscape position changes, which makes spatially predicting nitrate concentrations across the field a moving target.
- Soil geography studies the formation and distribution of soil on the Earth’s surface.
- Soil maps are representations of the spatial variability of soil at one specific moment in time.
- Dynamic properties (which change over time) become challenging to represent on a static map.